Sinter brazing has become a significant tool in the manufacture of complex powder metal components. The primary issue associated with this technology is holding the filler metal at the joint while sintering. The capillary forces of the porous base material and the braze joint, both compete for the filler metal. In cases where the filler metal is pulled into the compact and away from the joint, weak, or no bonding may result in a defective component. Only by understanding the variables that influence the control of the filler metal can a defined process be implemented to ensure optimal quality. The variables investigated in this study are: compact density, atmosphere chemistry, and the presence of flux in the filler metal.
Introduction
Sinter brazing has become a necessary means of manufacturing many complex powder metal shapes. Although the compaction technology has advanced substantially over the years, the ability to bond two components together while sintering has enabled the industry to broaden its capabilities and remain competitive in many markets.
The primary issue with the brazing of two powder metal components, in either the green or sintered states, is the competition between the capillary effect that draws the filler metal into the gap and the capillary effect produced by the porosity of the compact that results in the filler metal being pulled away from the gap and into the part. If this filler metal is pulled into the part, the bond may be compromised or not form at all.
In this work, we review the key fundamentals of brazing and apply them in such a way as to better control the flow and retention of the filler metal at the sinter braze joint. This is achieved by reviewing the key variables and their effect on the wetting of the filler metal. The sintering atmosphere, flux content of the filler metal, and the density of the components are all important to the control of the filler metal.
Brazing fundamentals
The fundamental driving forces for the formation of a good braze joint are the wetting of the base metal by the filler metal and the capillary force that results and pulls the filler metal through the braze gap.
Capillary Force =
Where ¡ is the surface tension, Q is the contact angle, and r is the radius of the capillary.
There are a few key steps that must be followed to produce a good braze joint:
Surface Preparation
- When the filler metal melts, the wetting of the filler metal is strongly affected by the cleanliness of the surface.
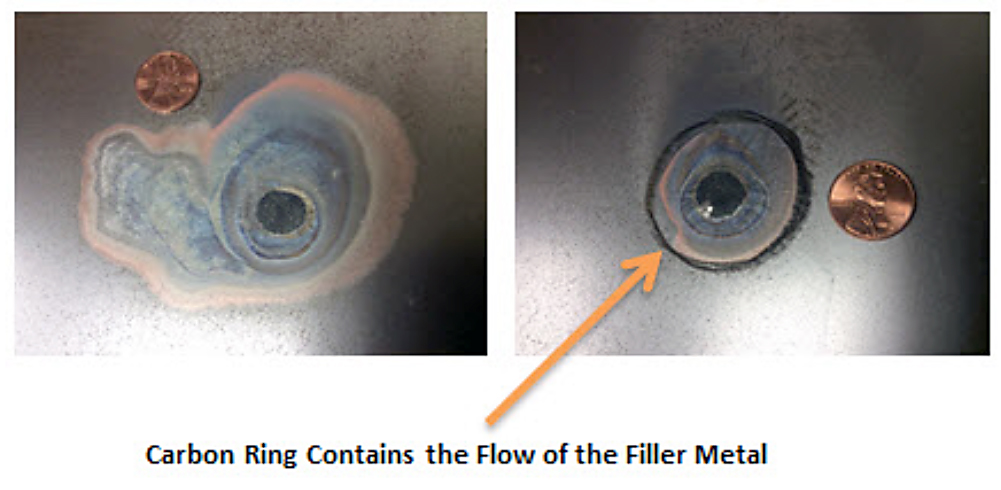
Figure 1 shows the effect of a carbon ring on the flow of filler metal (AB-72) on a carbon steel plate. If the surface is not free of dirt, oxides, and oils, the filler metal will not wet the base metal and will not be pulled into the braze joint.
Braze Joint Design
- Proper braze joint design is critical to achieving the desired strength in the component. The total length of the braze joint should be three times the thickness of the thinnest cross section being joined. This will ensure that a good braze will result in a joint that is stronger than the base metal. (Figure 2)
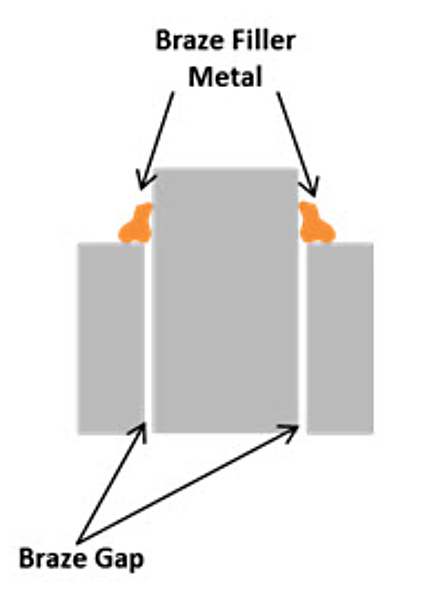
- The gap, through which the filler metal is pulled via the capillary force and is a strong function of the gap r, must be 0.05 mm (0.002”) to 0.20 mm (0.008”) wide at the brazing temperature. This means that the thermal expansion of the components must be considered when designing the joint.
Furnace Atmosphere
- All metals contain oxides on their surface. If these are not removed, the filler metal cannot alloy with the base metal. Hence, the oxides must be removed from the surfaces of the base metal and filler metal prior to the filler metal melting. This can be accomplished by using a flux (typically an acid or other material that will remove/react with the oxide layer to produce a clean metal surface.) or a reducing furnace atmosphere. Unlike a flux, the reducing furnace atmosphere will not leave a residue that must be removed post brazing. Some typical dew points of reducing atmospheres are -51oC (-60oF) for stainless steels and -35oC (-30oF) for carbon steels.
Time and Temperature
- The time for a filler metal to flow into a clean, properly designed braze joint and form a good braze is three to five minutes at the brazing temperature that is above the liquidous temperature of the filler metal.
Sinter brazing
Sinter brazing is a manufacturing technique used to produce complex shapes from powder metal that cannot be produced directly from the compaction press. Multiple shapes are produced using current pressing technology. These shapes are then assembled in the green state, or some may be presintered, and a filler metal is placed in or near the adjoining surfaces of the shapes to allow for brazing to occur simultaneously with the powder metal sintering process.
Common to sintering and brazing, the metal particles and surfaces must be free of dirt and oils. The hydrogen in the furnace atmosphere then reduces the oxides on the surface of the metals to produce clean particles and surfaces for sintering and brazing. As the components continue to travel through the sintering furnace and heat up to the sintering temperature, the filler metal melts and begins to wet the surface of the component surfaces; however, this is where the most common issue related to sinter brazing arises. Not only is the filler metal able to wet the surfaces that are to be brazed together, but the pores within the compacted components also compete for the filler metal. The surface tension between the filler metal and the base metal, along with the capillary effect produced by the small pores of the compact, pull the filler metal away from the braze joint and compromise the bond. This compromise may be a reduced amount of joint filling to no bonding at all.
If the wetting characteristics of the filler metal can be controlled, the retention of the filler metal at the brazing joint can be maintained and an improvement in the bonding and overall strength of the assembly may be maximized. For many years, manufacturers have added sulfur to the compact mix and manganese to the filler metal to retard the wicking of the filler metal into the pores of the compact. Unfortunately, sulphur is not always a desired additive. For this reason, current techniques use the addition of carbon-monoxide-containing atmospheres as a sintering atmosphere or as an addition to the sintering atmosphere. The impact of and mechanisms associated with the carbon monoxide addition were not clear; however, many swear by this approach. In this work, along with the influence of flux and density, the impact of and the mechanism associated with the addition of the carbon monoxide as it relates to controlling the wetting of the filler metal and its retention at the braze joint is investigated.
Experimental procedure
Discs of F-0000 were compacted to densities of 6.0 g/cc, 6.2 g/cc, 6.8 g/cc, and 7.0 g/cc. The discs had a dimension of approximately 7.62 cm (3 inches) in diameter by 2.54 cm (1 inch) thick.
Two filler metal chemistries were pressed into 1.5-gram pellets. One of the chemistries was a standard AB-72 material. The other filler metal chemistry did not contain a fluxing agent.
The pellets were then placed on top of the discs and sintered in two different furnace atmospheres, one atmosphere contained hydrogen, nitrogen, and carbon monoxide, and the other contained only the hydrogen and nitrogen.
The discs were then sectioned, polished, and etched with 2 percent Nital etchant to reveal the infiltration of the filler metal into the compact. Image J software was used to measure the infiltration area.
Results
As can be seen in Figure 3, increasing compact green density results in a decrease in the amount of filler metal that flows into the powder metal compact. This is to be expected since there is a 16.7 percent difference in the relative pore fraction when comparing a 7.2 g/cc compact and a 6.0 g/cc compact.
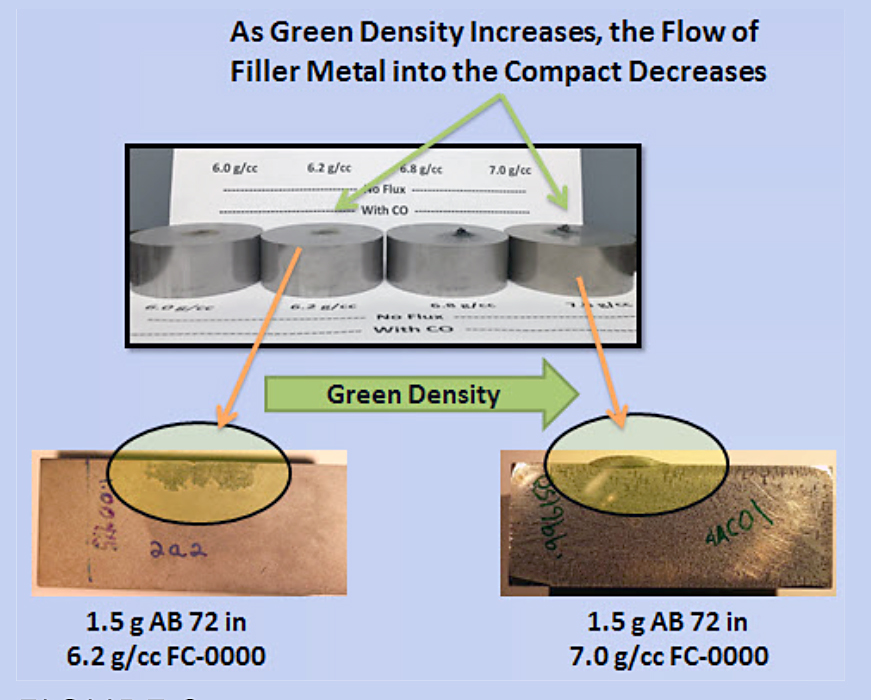
Flux is often added to the filler metal to help to clean the surface of the base metal and enhance wetting; however, in this application, the flux not only cleans the braze joint surface but also the particle surfaces inside of the pores of the compact. The result is an enhance wetting of the pore surfaces and more filler metal being pulled from the braze joint (Figure 4).
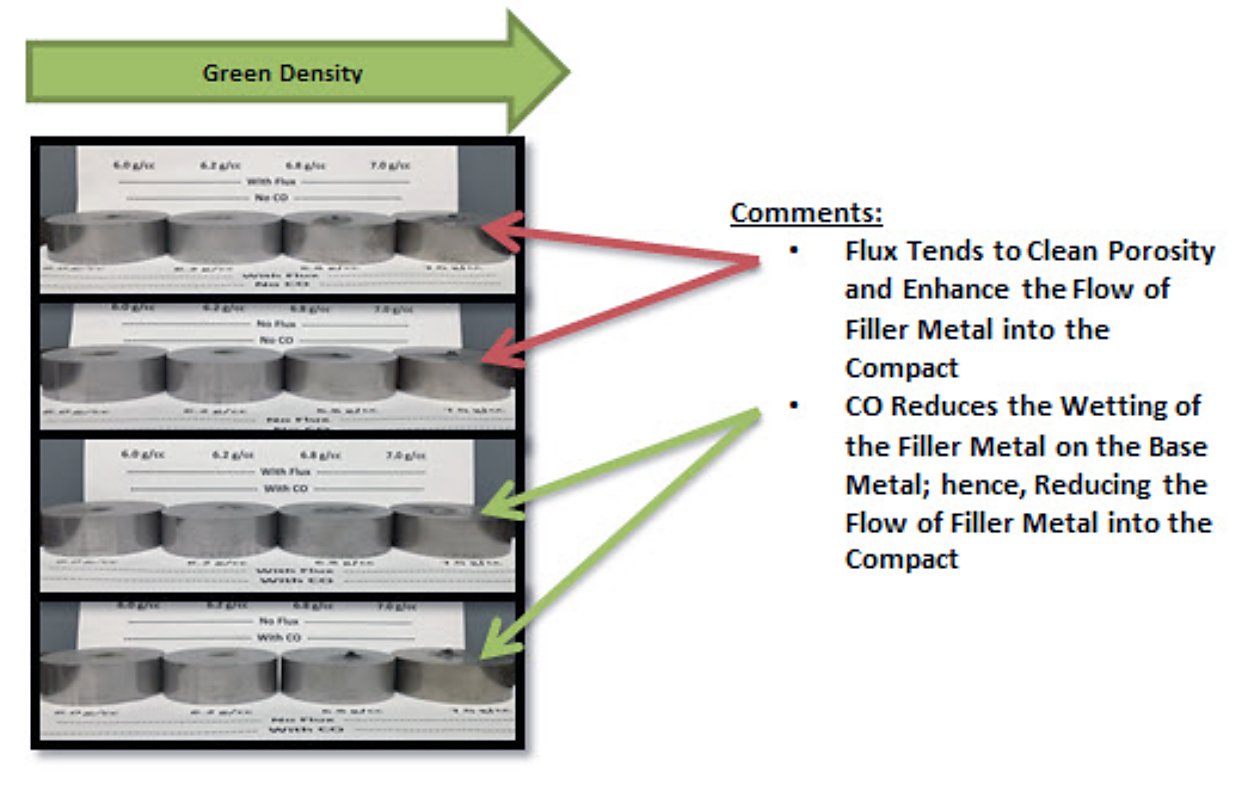
As the powder metal compact exits the preheat section of the furnace and enters the high heat section of the furnace, the compact temperature is in the range of 900oC (1,650oF) and 1,035oC (1,900oF), carbon monoxide results in the carburization of iron and steel at these temperature [1]. Since the amount of time that the product is in this condition is small, the carburization will be a small layer on the surface of the exposed particles on the surface of the material and inside of the pores. This surface contamination reduces the wetting of the pores by the filler metal and results in less filler metal being pulled from the braze joint (Figure 4). Figure 5 shows that, although this material is a F-0000 and contains no carbon, the surface of the particles in along the very edge of the compact were carburized. The particle hardness on the surface is higher, and the microstructure shows the presence of carbon on the surface of the particles.
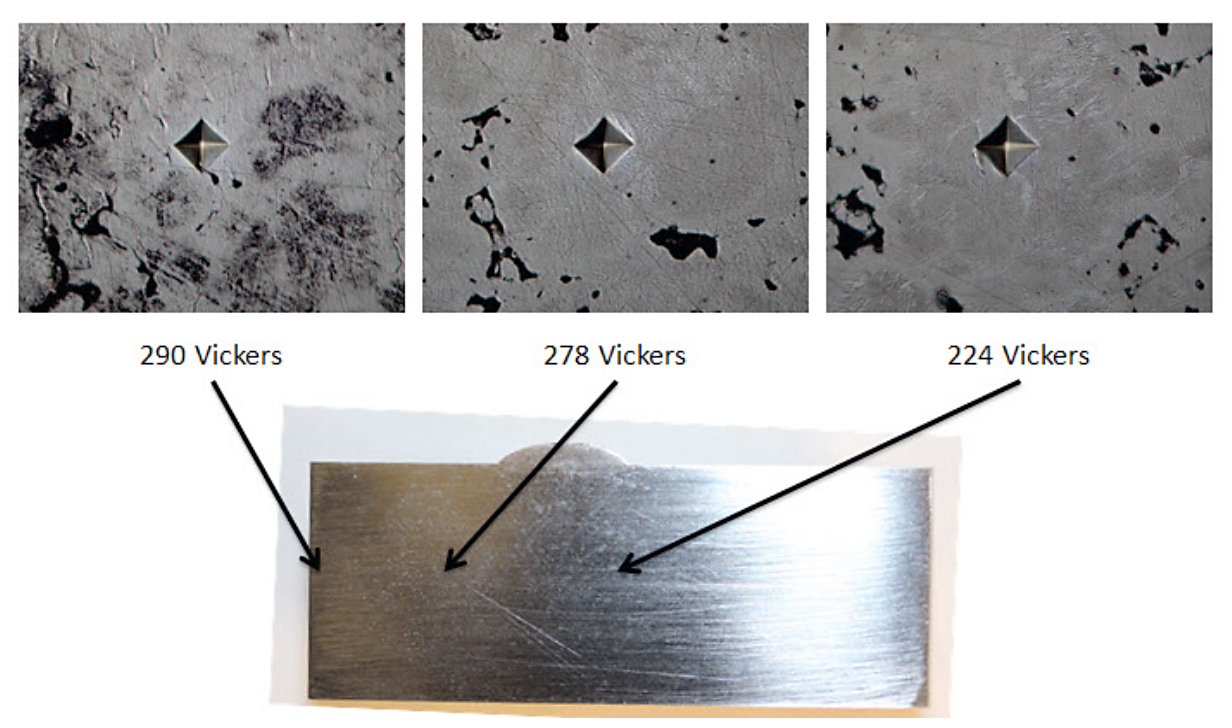
In comparing the overall impact of the variables (Figure 6), an interesting phenomenon was observed. In the high-density region of the graph (purple), the effects of the flux, carbon monoxide, and density were consistent with what was expected. In the lower density section of the graph (green), the control of the filler metal wetting was not influence by the wettability of the filler metal on the base metal.
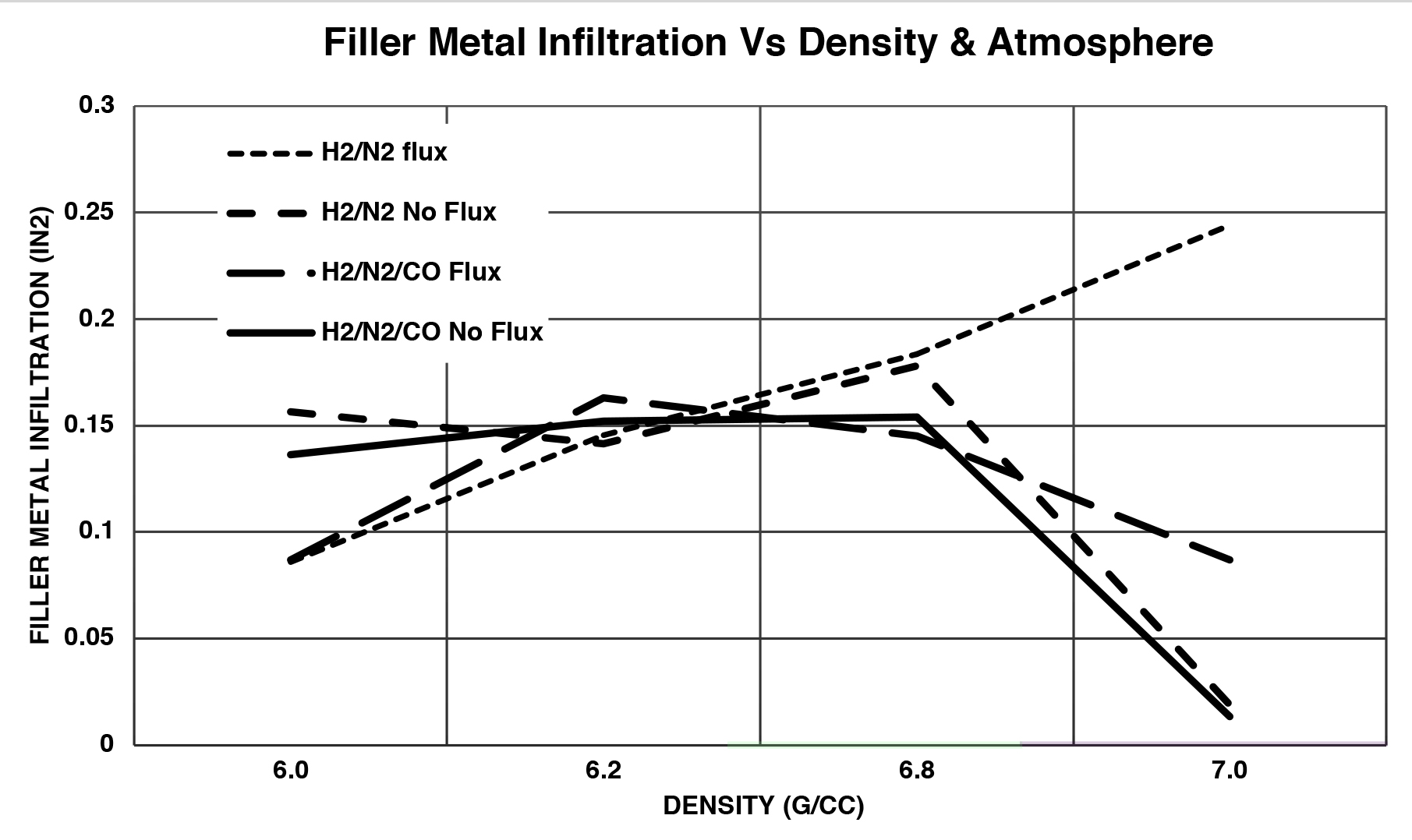
To better understand what is shown here, one must consider the relationship between the force due to surface tension that would want to pull the filler metal into the pores of the compact, and the force due to gravity, resisting the movement of the filler metal flow.
Figure 7 illustrates that as the pore size becomes larger, lower density of compact, there reaches a point where the force due to gravity will cause the filler metal to fall and not completely fill the pore.
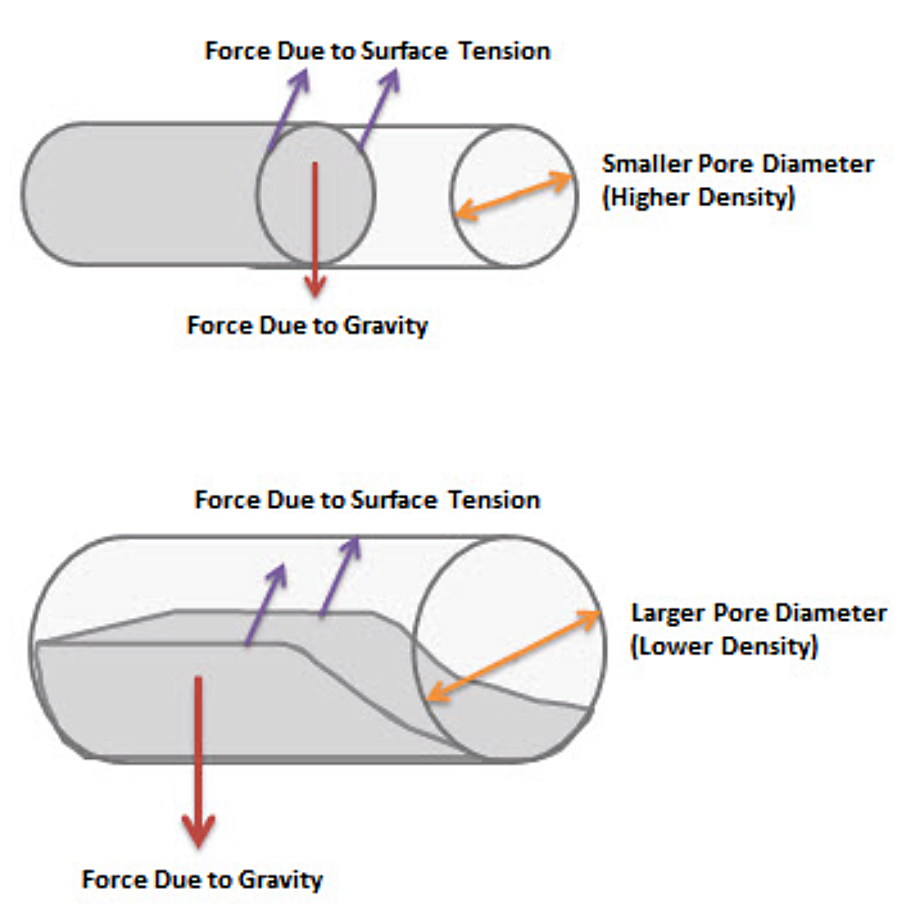
The result is that the influence of the altered surface tension is reduced and limits the ability of the producer to influence the wetting and flow of the filler metal with flux or carbon monoxide. For this reason, many producers find that they have more consistent results and better quality of sinter brazed products that are 6.9 g/cc and above.
Conclusion
The control of the filler metal at the braze joint of a sinter brazed product has significant impact on the quality and yield of complex shapes that cannot be produced by current conventional compaction technology. Maintaining the presence of the filler metal at the sinter braze joint is necessary, or the components will not be bonded. However, the porosity of the powder metal compact results in capillary forces that tend to wick the filler metal away. By pressing the compacts to a density greater than 6.9 g/cc, removing the flux from the filler metal, and adding carbon monoxide to the sintering furnace atmosphere, the capillary action of the pore can be reduced, and the flow of the filler metal at the joint can be controlled to produce a better bond.
References
- Heat Treater’s Guide, 2nd Edition, pg. 47, ASM International, 1995
- Bear, et al, Applying Brazing Fundamentals to the Sinter Brazing Process, May, 2017, American Welding Journal, pg. 44.
- Brazing Handbook, 5th ed. 2007, Miami, Fla.: American Welding Society
- Bear, K., and Feldbauer, S., Fundamentals of Brazing Applied to Sinter Brazing, Presentation, Special Interest Session, PowderMet 2016, Boston, MA.
Reprinted from Advances in Powder Metallurgy & Particulate Materials — 2017, ISBN 978-1-943694-15-0, © 2017 Metal Powder Industries Federation, 105 College Road East, Princeton, New Jersey, USA.