Low pressure carburizing (LPC) is the technology of choice for the precise carburizing of high-performance gearing [1]. To achieve absolute process repeatability and the highest quality outcome, it should come as no surprise that one of our goals is to fix as many of the process parameters (e.g. hydrocarbon gas type, pressure, flow rate, etc.) as possible (Figure 1). Selecting acetylene as the hydrocarbon gas of choice helps us achieve this.

Historical perspective
The history of vacuum carburizing is a fascinating one. The process was invented in late 1968 and subsequently patented (U. S. Patent No. 3,796,615, U. S. Patent RE 29,881) by Mr. Herbert W. Westeren, Director of Research and Development for C. I. Hayes, Inc., Cranston, RI. Commercialization followed in early 1969 (Figure 2).
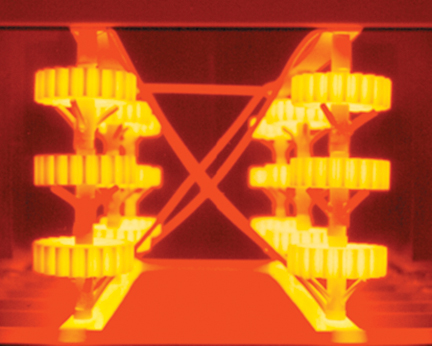
Full acceptance of the process by industry, however, involved three decades of work and contributions from all over the world including the discovery and patenting of acetylene technology in the former Soviet Union (USSR Patent No. 668978) by V. S. Krylov, V. A. Yumatov and V. V. Kurbatov in 1977 and culminating in the application and patenting (U. S. Patent No. 5,702,540) of acetylene based carburizing by Mr. K. Kubota, JH Corporation (formerly Japan Hayes Corporation), Nagoya, Japan. Since that time, a significant number of individuals and companies have made patentable inventions that have helped advance the technology [1].
The worldwide technology advances needed to make LPC a viable technology included:
- Improvements in the design and construction of vacuum furnaces;
- Development of low pressure (< 20 torr) carburizing methods;
- Process optimization – especially the selection of hydrocarbon gas;
- Development of optimized gas injection methods and flow/pressure controls;
- Creation of empirical data bases and design of process simulators;
- Development of high pressure gas and oil quenching technology;
- Availability of low cost carburizing alloys specifically designed to take advantage of vacuum carburizing – including high temperature capability.
Modern-day carburizing installations (Figure 3) have taken advantage of these developments.
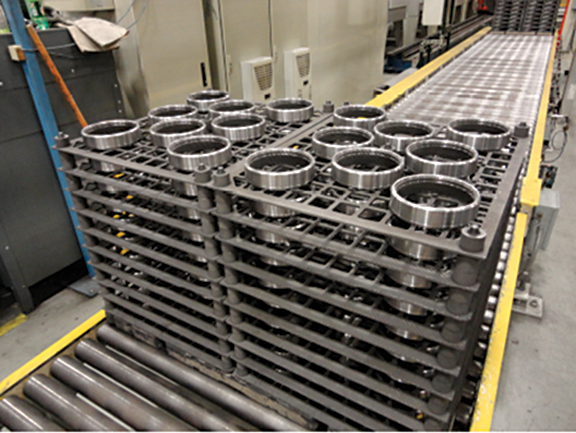
The LPC process explained
LPC is a recipe-controlled boost/diffuse process. By contrast, atmospheric gas carburizing is controlled via carbon potential. In vacuum carburizing, process-related parameters such as temperature, carburizing gas-flow, time, and pressure are adjusted and controlled to achieve the desired case profile in the parts.
One method of recipe development involves solving the following three (3) equations:
- D = k√t
- c = rt
- t = c + d
Where:
- D = effective case depth (50 HRC equivalent)
- k = carburizing constant (c.f. Table 1)
- t = total time, in hours
- c = carburizing time, in hours
- r = boost/diffuse ratio (c.f. Table 1)
- d = diffusion time, in hours
Simulation programs are available from any number of suppliers of vacuum carburizing equipment and are designed to create recipes and test scenarios for process development. These programs are based on a mathematical description of the carbon dissociation and adsorption of the carbon at the surface of the parts and equations, which describe the diffusion of the carbon into the material. While the carbon transport to the surface in low pressure vacuum carburizing differs significantly from that in atmospheric gas carburizing, the same diffusion laws apply for the carbon transport within the material.
Typical input parameters of the software included:
- Material
- Carburizing temperature
- Targeted carburizing depth
- Targeted surface carbon content
- Surface carbon content limit
- Load surface area
The simulation program can be used in two modes. The first mode allows one to enter a desired surface carbon content and case depth. Then the program calculates the required recipe consisting of different carburizing and diffusion pulses (Figure 4). Furthermore the program shows diagrams of carbon flux versus time and carbon profile versus time and distance from surface.

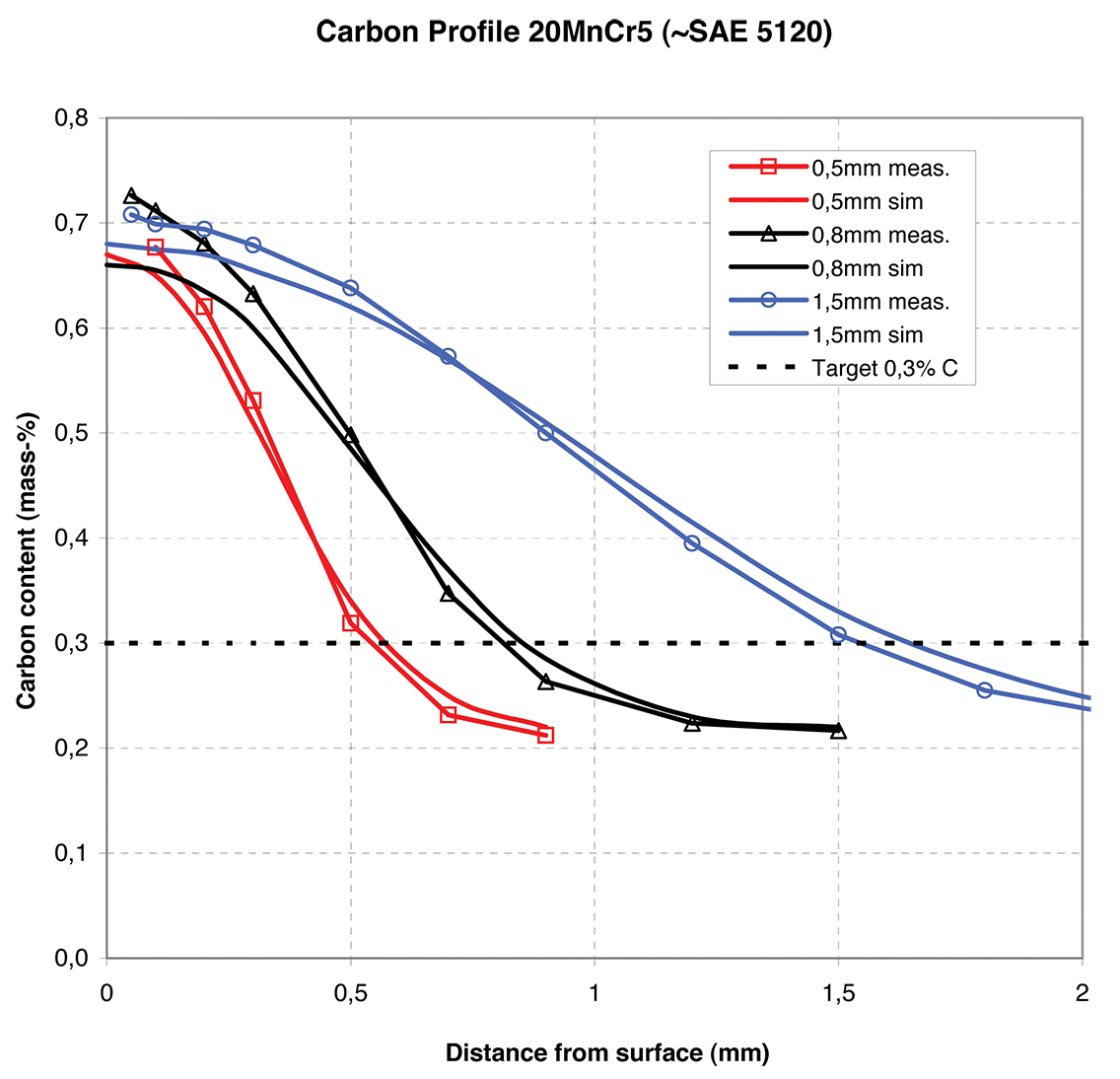
The second mode is to enter a certain recipe consisting of carburizing and diffusion pulses and the program calculates the resulting carburizing profile. The first mode is generally preferred. These simulations have been found to be quite accurate (Figure 5). The simulated carbon profile is very similar to the measured carbon profile. Some simulation programs also include a quenching module for calculation of the case hardening depth instead of the carburizing depth.
The use of a simulation program to create a carburizing recipe is well accepted for LPC applications in all manufacturing industries including automotive and aerospace. Using these simulators, a test load is run and the parts are checked for correct case profile. Normally, the achieved case profile is within the specified range. If not, the parameters are adjusted slightly and a second cycle is run. Since the LPC process offers consistent case uniformity, it might be advantageous to perform additional simulation runs so as to adjust the case depth towards the lower end of the case-specification. This is done to reduce the overall process time thereby increasing the productivity of the system. As soon as the required profile is achieved, the recipe is fixed and not changed.
Furthermore the simulation program is a powerful tool to achieve the wanted microstructure after vacuum carburizing. The program shows the formation of the carbon profile as a function of time for different surface distances. Therefore it is possible to create recipes that meet microstructural specification, i.e. the absence of large quantities of carbides or avoidance of excessive amounts of retained austenite.
A further result of the simulation program is the calculation of the correct hydrocarbon flux, which depends on the actual load surface area and the carbon yield of the carburizing gas that is used. The carbon yield defines the amount of carbon transferred into the parts in relation to the amount of carbon supplied to the treatment chamber by injecting the carburizing gas. For acetylene the carbon yield is in the range of 60% to almost 80%. This tool allows the user to reduce the amount of carburizing gas to a minimum, which is both economically and ecologically beneficial.
As the chemistry of the materials to be carburized has an influence on the carburizing profile to be achieved, it is possible for a user to enter the exact chemical composition of their own material into the program and store this data in the already existing material data bank.
Hydrocarbon choices
Over the years a number of hydrocarbon gases and liquids (Table 2) have been used to supply a source of carbon for LPC. While all of these choices are possible, acetylene and acetylene mixtures have become the dominant choice in the industry.
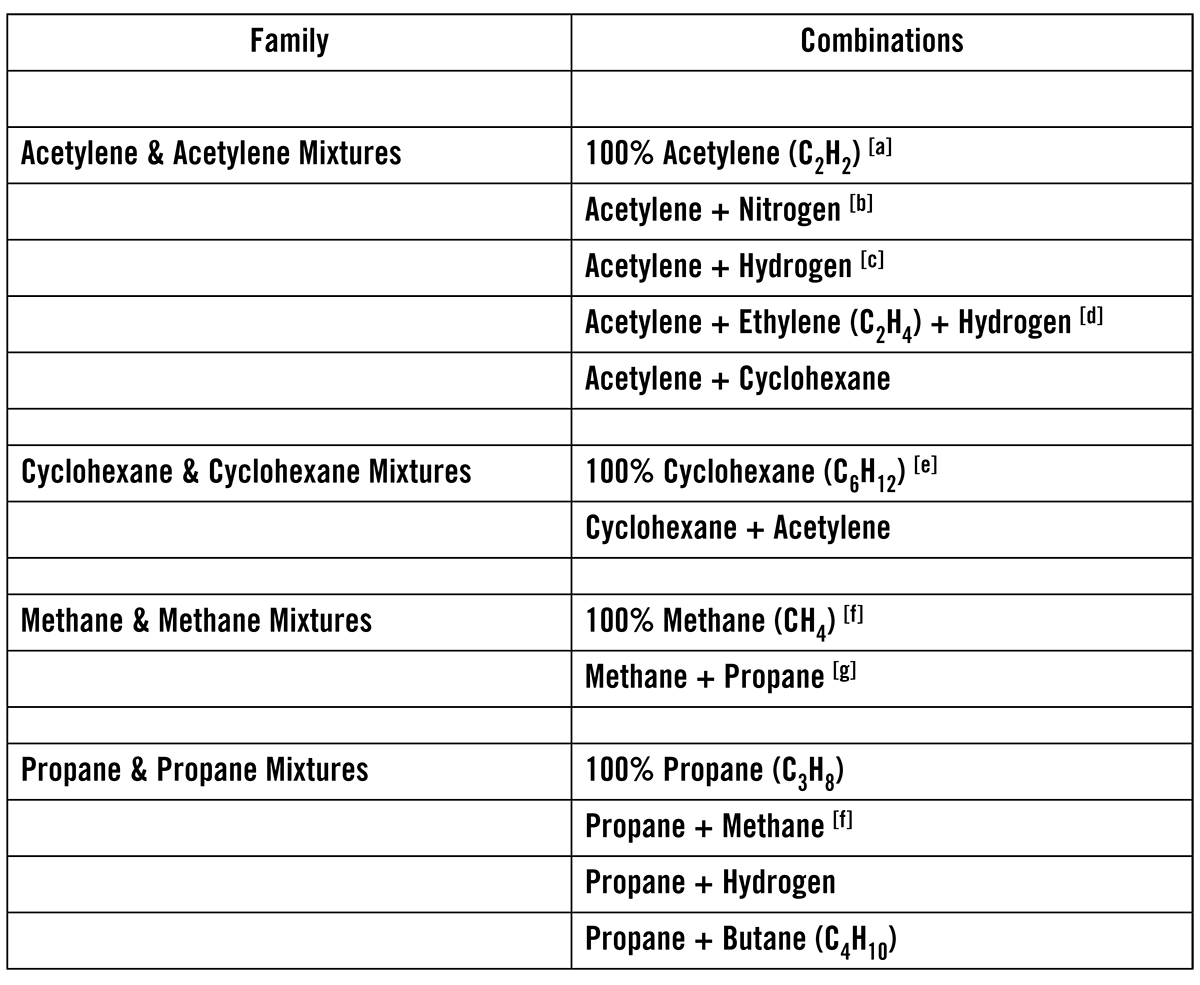
Notes:
- DMF Acetylene (without acetone) preferred, though not mandatory
- Typical dilutions up to 50%
- Typical dilution 7:1 (US Patent 7,514,035 Solar Atmospheres Inc.)
- Typically ratios of acetylene to ethylene to hydrogen are 3:2:1 or 2:2:1 (US Patent 7,550,049 SECO/WARWICK Corporation)
- Cyclohexane (US Patent 7,267,793 Surface Combustion, Inc.)
- Temperatures above 955°C (1750°F) recommended unless plasma assisted
- Typical dilution: 40/60 to 60/40 (methane/propane)
Why acetylene?
Acetylene is a catalytically decomposable hydrocarbon, which essentially means that it will break down into its elemental constituents (Equation 1, Figure 6) in the presence of an iron catalyst. Other hydrocarbons (e.g. methane, propane) are thermally decomposable, which means that they will break down immediately upon entry into the hot zone of the vacuum furnace negating the ability of the carbon to react with the surface of the steel and creating unwanted hydrocarbon byproducts (Figure 7) and ultimately contaminating the hot zone. In addition, acetylene has a higher average carbon flux, about 150 g/m2-h, then other hydrocarbons. For these technical reasons, acetylene is a strong candidate for the preferred hydrocarbon gas choice.
(1) C2H2 —> 2C + H2
Acetylene decomposition – RGA analysis
In general, carburizing gases must have high purity (99.95% or better) and have the ability to be effective at low (< 20 torr) pressure. The use of high pressures coupled with impure gases (i.e. the presence of so-called “heavy” hydrocarbons) has in the past resulted in the formation of excessive deposits of soot and tar (Figure 8) and unacceptably high equipment maintenance. Acetylene avoids these issues and dramatically reduces maintenance time and cost.

DMF Acetylene
Gas consistency plays an important role in the hydrocarbon choice for LPC. Chemically produced acetylene is transported in a solvent, either acetone or DMF (dimethyl formamide). DMF has a boiling point about 100˚C (212˚F) higher than acetone, and acetylene and similar solubility (Table 3). Thus DMF is less likely to volatilize and enter the vacuum furnace reducing the risk of introducing oxygen (leading to concerns over intergranular oxidation) or other unwanted constituents into the carburizing process.
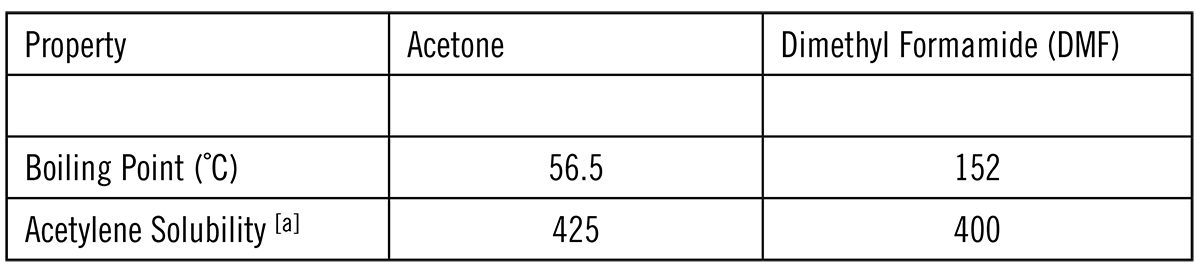
Application example
Full production loads (Figure 9) of several types of SAE 8620 transfer pinion gears and clutch hubs (Figure 10) were run using two (2) different carburizing methods (gas atmosphere and LPC). In the case of the acetylene vacuum carburized gears, oil and high gas pressure quenching methods were employed. In the case of the atmosphere-carburized gears, traditional plug quenching methods were used.
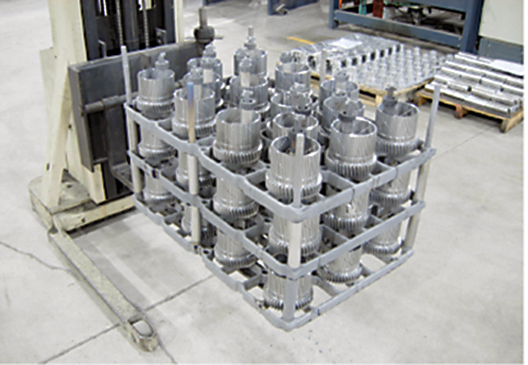

Low pressure carburizing was performed at 960°C (1760°F) for 3.34 hours (boost/diffuse time) with acetylene (2200 nL/h, 10.5 mbar) followed by either oil quenching (70% agitator speed) or high pressure gas quenching (11 bar, nitrogen). Targeted effective (50 HRC) case depth was 1.25 mm (0.050”) with a surface carbon content of 0.72%C. Gas quenching utilized four (4) changes in speed and pressure made through the critical transformation range of the material.
Atmosphere carburizing (Endothermic gas, natural gas additions) was performed at 960°C (1760°F) for 4.0 hours with a carbon potential of 1.3% followed by slow cooling, reheating to 830°C (1525°F), stabilizing and plug quenching (45 seconds) in oil. Targeted effective (50 HRC) case depth was 1.25 mm (0.050”) with a surface carbon content of 0.80 -0.90%C.
All gears were subsequently tempered at 150°C – 175°C (300°F – 350°F) for two (2) hours at temperature.
The result of processing this family of gears found that:
- Low pressure vacuum carburizing in combination with high pressure gas quenching produced the most consistent repeatability.
- The degree of dimensional change is capable of being compensated for in standard post manufacturing processes.
- Low pressure vacuum carburizing in combination with high pressure gas quenching allowed for the replacement of atmosphere carburizing and plug quenching on all gears in this family.
- The depth of high hardness (> 58 HRC) was greatest in the low-pressure vacuum carburized samples.
- The root-to-pitch line case depth ratio was 93% for vacuum carburizing compared to 63% for atmosphere carburizing.
- Atmosphere carburizing resulted in unacceptable levels of retained austenite.
- Gear charts indicated an average movement of 0.08 mm (0.003”). The involute form remained intact after low pressure vacuum carburizing and gas quenching, as did the lead on the gear teeth and splines.
Conclusion
While many choices for hydrocarbon gases are possible in LPC, and although special circumstances may necessitate an alternative choice, acetylene and acetylene mixtures have clearly separated themselves as the preferred hydrocarbon choice from a process, equipment and quality standpoint. In the heat treatment process, every choice matters. Remember that the right hydrocarbon choice will save time and money, as well as improve the final product.
References
- Herring, Daniel H., Vacuum Heat Treatment, BNP Custom Media Group, 2012.
- ASM Handbook, Volume 4: Heat Treating, ASM International, 1991, p. 350.
- Jones, William R., Low Torr Range Vacuum Carburizing in an Experimental Vacuum Furnace, IIT/TPTC Vacuum Carburizing Symposium, November 2004.
- Esper, Bob, Acetylene: The Right Carbon Source for Low-Pressure Carburizing, Industrial Heating, 2009.
- Otto, Frederick J., and Daniel H. Herring, Improvements in Dimensional Control of Heat Treated Gears, Gear Solutions, June 2008.