Large gear components can be offered in many applications such as marine, wind power, steel rolling mills, power plants, transportation, railroad, aircraft, cement crushers, mining, and oil industry applications. There are three important surface hardening methods, as seen in Figure 1, used to improve and expand the technical use of gear components. Design and material engineers must decide which hardening method to use. Case hardening is normally the first choice because of the highest load capacity. However, case hardening also poses challenges that must be acknowledged. Therefore, it is good to know that there are three options for very large components. So first, let´s compare these methods.
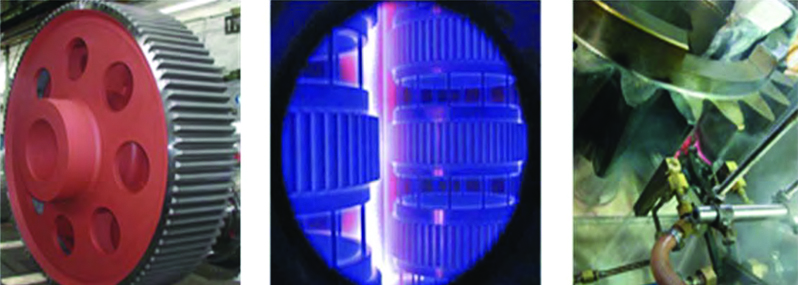
Three Methods Compared
Case hardening is normally carried out at temperatures between 880°C to 980°C for carburizing, and 780°C–860°C for hardening. The standard procedure is gas carburizing. By diffusion of carbon into the surface and quench hardening, the process produces a strong hard surface layer of martensite of up to 10 millimeters. This thermochemical method adds defined quantities of carbon to the workpiece by using a carbon enriched gas (i.e. methane (CH4) or propane (C4H8)). After carburization, the components are hardened and tempered to the required surface hardness to relieve internal stresses. In addition to a high surface hardness (max 850 HV) and abrasion resistance, the heat-treated workpieces exhibit good reverse bending and fatigue strengths due to residual compressive stress. Specific time and temperature variations in the carburizing, hardening, and tempering processes can be introduced to optimize the material properties and minimize the changes in dimensions associated with the respective charging techniques—hereto lies the art of hardening. Nitriding treating temperatures range from 500°C–580°C for gas nitriding, and from 400°C and up for plasma nitriding and plasma nitrocarburizing. Nitriding is a method for enriching the surface layer of ferrous materials with defined quantities of nitrogen or, in the case of nitrocarburizing, of nitrogen and carbon. This not only enhances the hardness, but also the abrasion resistance, fatigue strength, corrosion resistance, and antifrictional properties. Furthermore, there are no microstructural transformations from austenite to martensite, so high dimensional stability is ensured.
Normally, nitriding penetrates to a maximum depth of 0.8 mm. “Profundinieren”, a deep nitriding method developed by Dr.-Ing. Helmut Reese, penetrates to depths exceeding 1.0 mm, depending on the material. Provided that the corresponding steels are used, non-deforming nitriding is in many instances a viable alternative to case and surface hardening. Nitriding steels are listed under DIN 17211 and EN 10085.
Surface hardening is carried out at treating temperatures 50°C–100°C above the material-specific hardening temperature. The heating can be done by flame, induction, laser, or electron beam. These processes produce a hard surface layer of martensite. These methods can also be used to harden large components or complex geometries. Induction or flame heating is applied to the heavily loaded areas (specific surfaces) of the workpiece until the respective hardening temperature is reached, after which the workpiece is quenched. Much experience is necessary for optimizing the methods and finding component-based solutions for both flame and induction hardening. Therefore, the evaluation and consistency of test samples is essential and is greatly enhanced by specific definitions of machine parameters. In summary, surface hardening is a technical and economical alternative to conventional case hardening in many instances. A side-by-side comparison of the three surface hardening methods can be seen in Table 1.

For the load capacity of a component, the important factors are hardness, case depth and core strength:
- If the load capacity of the gear is vital, case hardening is the first choice, even if the hardening distortion during nitriding is less.
- If Hertzian pressures are low, as in hydraulic cylinder applications, and low hardness depths are sufficient, nitriding is the first choice.
- Large hardness depths in a short time, partial hardening of large components, and flexibility are assets of the surface layer hardening
- The load capacities of the three hardening methods can be compared using Figure 2 where fatigue strength is measured against hardness.
- Because of the highest load capacity, case hardening is the first choice for the treatment of large transmission components.

Three main aspects for case hardening of big gear components have to be considered:
- Hardenability (material selection and geometrical influence)
- Weight (as much as necessary, as little as possible)
- Dimensional changes and distortion
Hardenability
Large components are normally quenched in oil. Cooling below the alloy Ms temperature through to the core often takes longer than one hour, depending on the size of the cross sections. During this time, the heat is conducting through the surface. Therefore, a steel must be selected, with its ferrite/pearlite nose as far to the right as possible. Otherwise, there will be a significant decrease of the surface hardness and case depth. The larger the cross-sections, the more it is necessary to utilize high-alloyed steels.
According to the experience at Reese, for large components with a cross section more than 100 mm, 18CrNiMo7-6 or 18CrNi8, or similar steel qualities with HH-alloying-scatter-band, must be chosen. The two time-temperature-transformation diagrams (see Figure 3 and Figure 4) exhibit the differences between a high alloyed and low-alloyed case hardening steel.

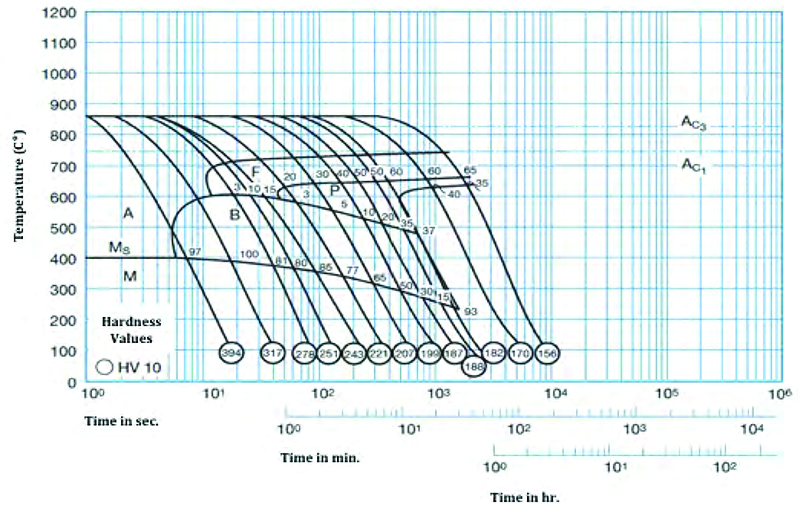
The alloying elements Cr, Ni, Mo, Mn and V increase the hardenability, and V, Ni and Mo additions increase toughness. If a steel is selected with insufficient hardenability, regardless of intensive quenching in oil with very good cooling and circulation, the result will be an unacceptable drop of hardness and hardness depth of large components. This hardness reduction is evident in gear parts especially below the pitch circle down to the tooth base, to a hardness of partially well below 52 HRC. The components can fail after a short period of time by pitting, flank fractures and tooth root fractures. Using the same alloyed steels from Figure 3, Figure 4, Figure 6, and Figure 7, display the relationship of hardness as a function of the distance from the quenched end. As seen, there is an obviously much larger decrease in hardness as the distance between the quenched end grows with the low alloyed case hardening steel. The graphs created are a result of the Jominy hardenability test (Figure 5).
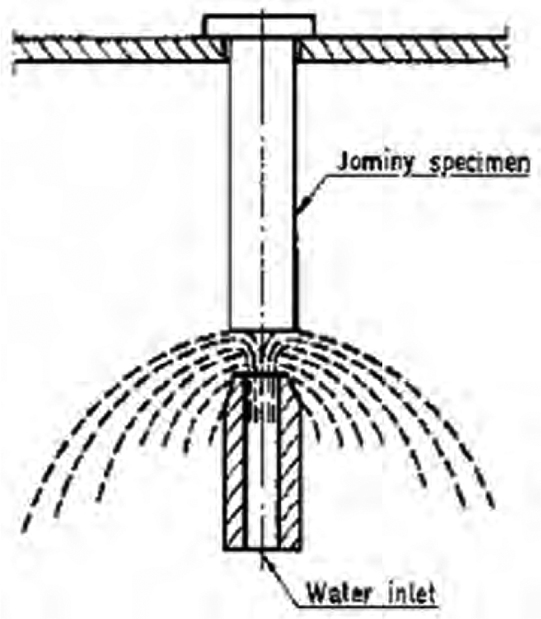
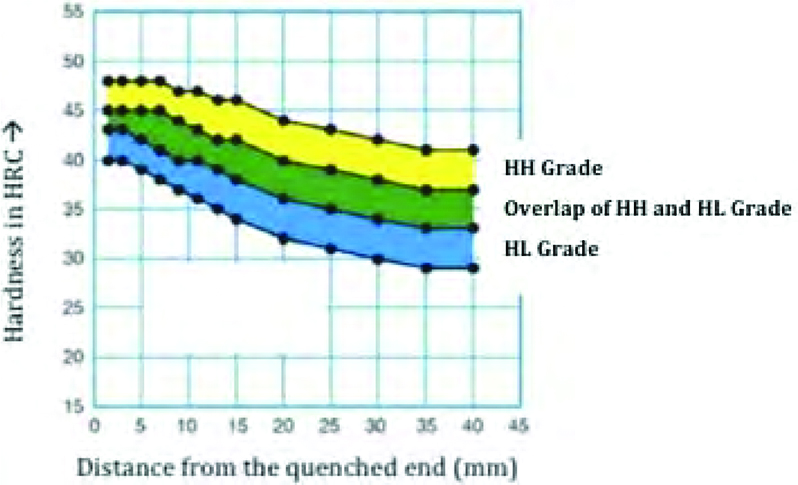

In the area of decreasing hardness to the tooth root, the CHD cannot be adequately modeled by the cylindrical geometry of a sample coupon. In particular, the geometric conditions and their influence on the cooling rates in massive components cannot be compared with small cylindrical test pieces. Case hardening results of the tooth base and tooth flank in comparison with a small cylindrical sample coupon are not a sufficient match of the carbon depth profile. The coupon sample should therefore be shaped such that it, in addition to a custom size, also has a greater geometrical resemblance to the tooth base. A comparison of flank and root-CHD and CHD of a ∅35 mm sample coupon can be seen in Table 2. A-F represents six companies that participated in a collaborative study FVA 501. Steel hardenability has a big influence on shape changes (shrinking and growing). Case hardening steel plants with modern computer controlled melting can specifically adjust the hardenability. It makes sense to require hardenability, grain size, and purity.

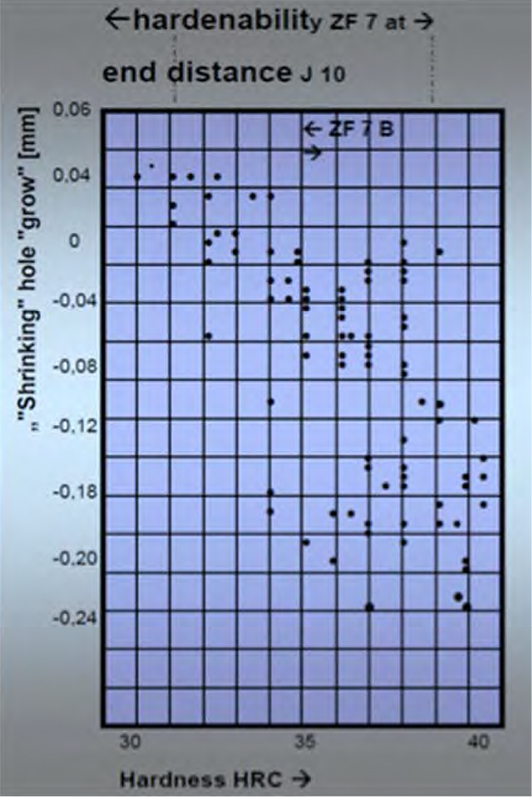
The cost is neutral for the specification for the lower 2/3 or the upper 2/3 band of hardenability. Even more favorable for the subsequent shape change, but subject to a cost surcharge, is the agreement about closer hardenability limits. Figure 8 displays possible shape change values when using case hardening steel as a function of the hardenability.
In conclusion, when selecting the material for large gear components, the geometrical influence must be taken into consideration and the following objectives must be achieved:
- A sufficient hardenability of the material must be chosen
- The material cross sections must be kept as small as possible
- The geometry must ensure dimensional stability.
Also:
- Grade steel (not “quality” steel) according to EN 10020
- Electromagnetically stirred and cooled with fan
- Fine grain steel
- Pre-hardening and annealing in oil or emulsion to estimate dimensional changes
Weight
The weight is critical in the first line for efficiency. Weight reduction should be sought in any case, but this must not be at the expense of stability. For large gear components, weldments have been proven to be efficient and are now standard.
Small and medium sized gears, as seen in Figure 9 and Figure 10, are usually made solid. Larger gears are usually designed as welded constructions.


The welds must be protected against carburization to avoid cracking during case hardening. See Figure 11, Figure 12 and Figure 13 for examples of larger gears containing weldments.
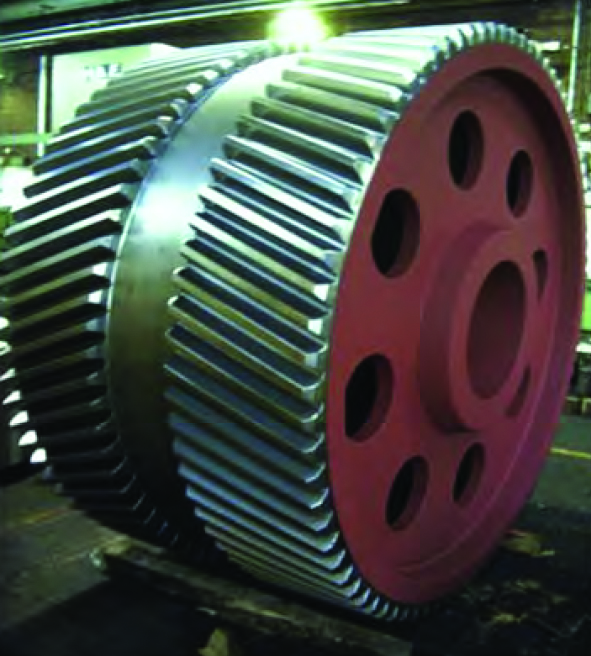
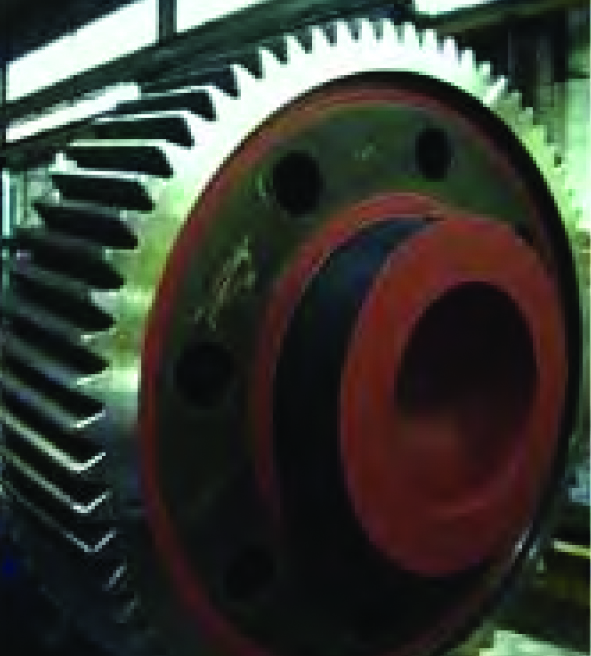

Welding is limited, however, by maximum preheating temperature of 350–400° C. Weldments are the design of choice today. They have good dimensional stability and reduce weight.
Pretreatment of the Material
According to our experience the following pretreatments have proven effective for big gear components and should be examined in detail on costs and benefits:
- 3-D forging (stretching and compressing with deformation to the core), leads to a more uniform part, reduction of segregation, reduction of pores, and refined structure to the core.
- Do not air cool after forging, but let the part temperature drop to 840°C. Then soak and harden in oil followed by tempering at 650°C.
- If this is not possible, then in addition to the forging shop, pre harden and temper at or higher that 840°C then oil quench and temper at 650°C to improve the toughness. One can draw conclusions about the dimensional change and distortion behavior by measuring before and after this process.
- Avoid the range of the irreversible temper embrittlement between 250–400°C during the entire process, i.e., rapid traveling across this range in all process stages. If possible cool from the annealing temperature of 650°C, in oil.
- Avoid microcracks, cavities, voids, dendrites and impurities to reduce the risk of hydrogen embrittlement and fatal delayed fractures.
- High precision of final workpiece dimensions
- Minimization of dimension failures and scrap
- Minimization of rework
Dimensional Change and Distortion
The reduction of dimensional change and distortion is becoming increasingly vital, depending on the size of a component. Both Figure 14 and Figure 15 display deformations from simulation of a gear by horizontal charging.

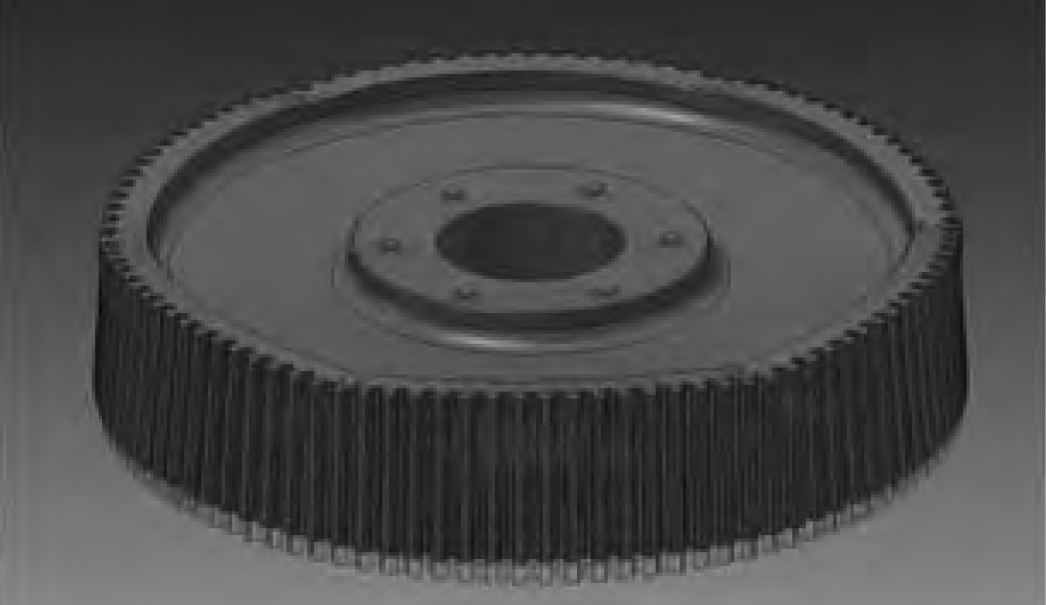
Dimensional change means growth or shrinkage. Distortion means:
- Tapering
- Ovality
- Axial runout
- Concentricity
- Dents of support tools caused by heavy weigh
A distortion of 1 per micron at a gear with a diameter of 1000 mm (40”) is only 1 mm (0.040”). A 1 per micron distortion for a 5000 mm (200”) diameter gearwheel will result in a 5 mm (0.200”) dimensional deviation. But 5 mm (0.200”) is often the whole case hardening depth CHD. That would mean that the total hardness would have to be ground away to keep the geometry. Therefore, the goal is to minimize distortion and to anticipate dimensional changes.

Factors that influence dimensional change and distortion as seen in Figure 16:
- Over 60% of the induced tensions are due to the design and manufacturing phase.
- Up to about 30% is based on the choice of the material and its quality
- Only about 10% of the influencing variables are born out of heat treatment.
Growth and shrinkage are dimensional changes caused by microstructural transformation and thermal stresses during the heat treatment process. These factors definitely determine the dimensional behavior of a workpiece, but remain mostly unavoidable during heat treatment. Distortions are caused by many factors. High residual stresses and differences in alloy concentration are among these factors as well as the choice of the material and its quality. Additionally, geometric asymmetry and non-uniform temperature distribution during the manufacturing process can result in distortions as well. However, they can be avoided by taking the appropriate measures in the steel order, construction and the workpiece production. During the heat treating process key aspects are plant engineering, process engineering and the type of charging (fixturing). These three factors however can outweigh the other factors. Thus, the hardening distortion gains a crucial importance for the success of the entire manufacturing process of big gear components.
Atmosphere control, temperature control, and quenching/cooling control are the key aspects of the technical features of a hardening plant. As seen in Figure 17, all aspects are thoroughly reviewed and considered before the heat treatment process can begin.

Positive Effects Due to Choice of Hardening Procedure
The differences in hardening procedures can have a great effect on the outcome of a gear. The process/environment a gear can be put through can very as seen in Figure 18. Table 3 compares direct hardening to other methods.
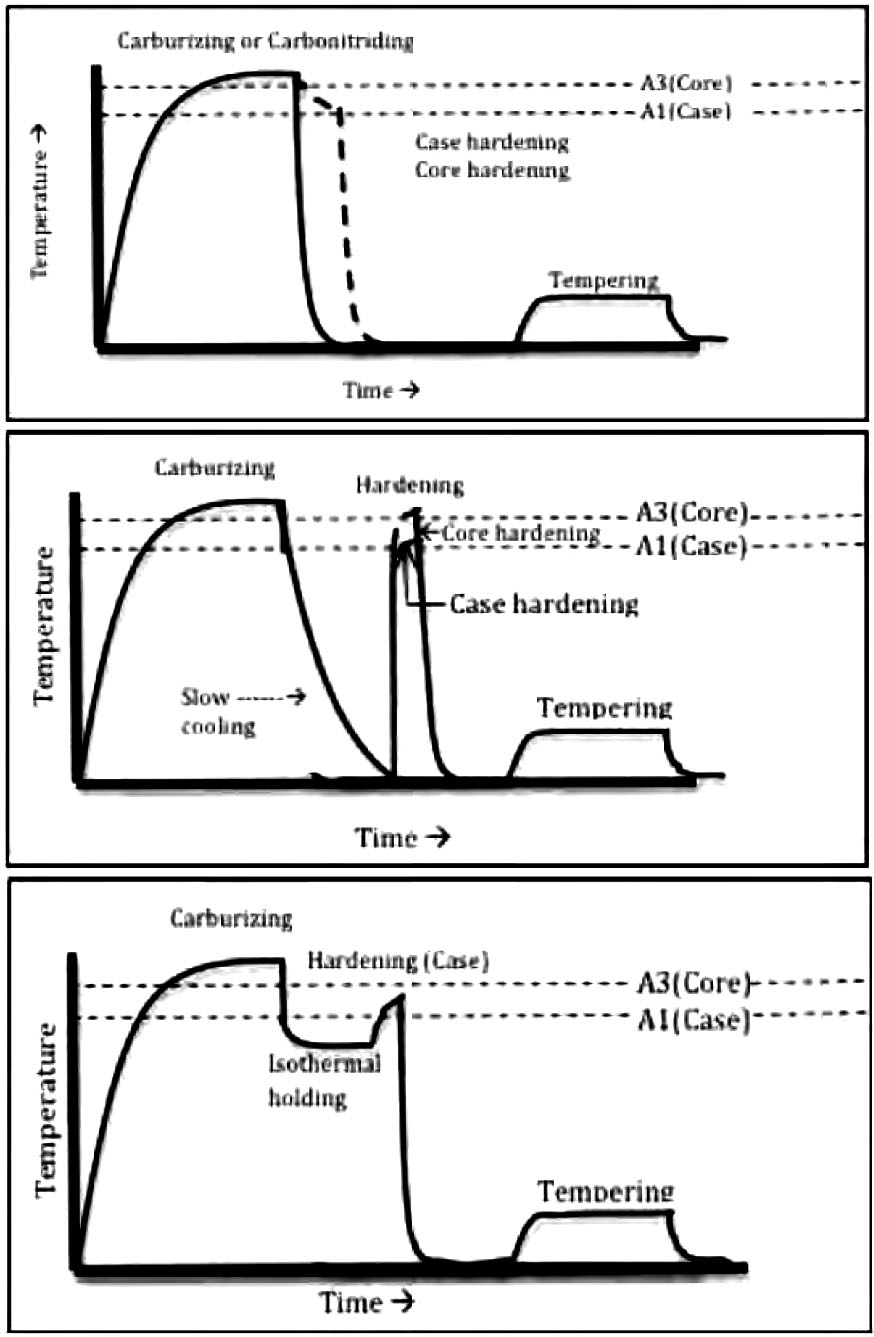

Positive Effect Due to Charging for Heat Treatment
Various charging (fixturing) methods can be seen in Figure 19, Figure 20, Figure 21, Figure 22, Figure 23, Figure 24. The possible charging methods include:
- Horizontal
- Vertical
- Hanging
- Standing
- Customized Charging Devices
- Batching Tools
- Custom-Made Charging Systems
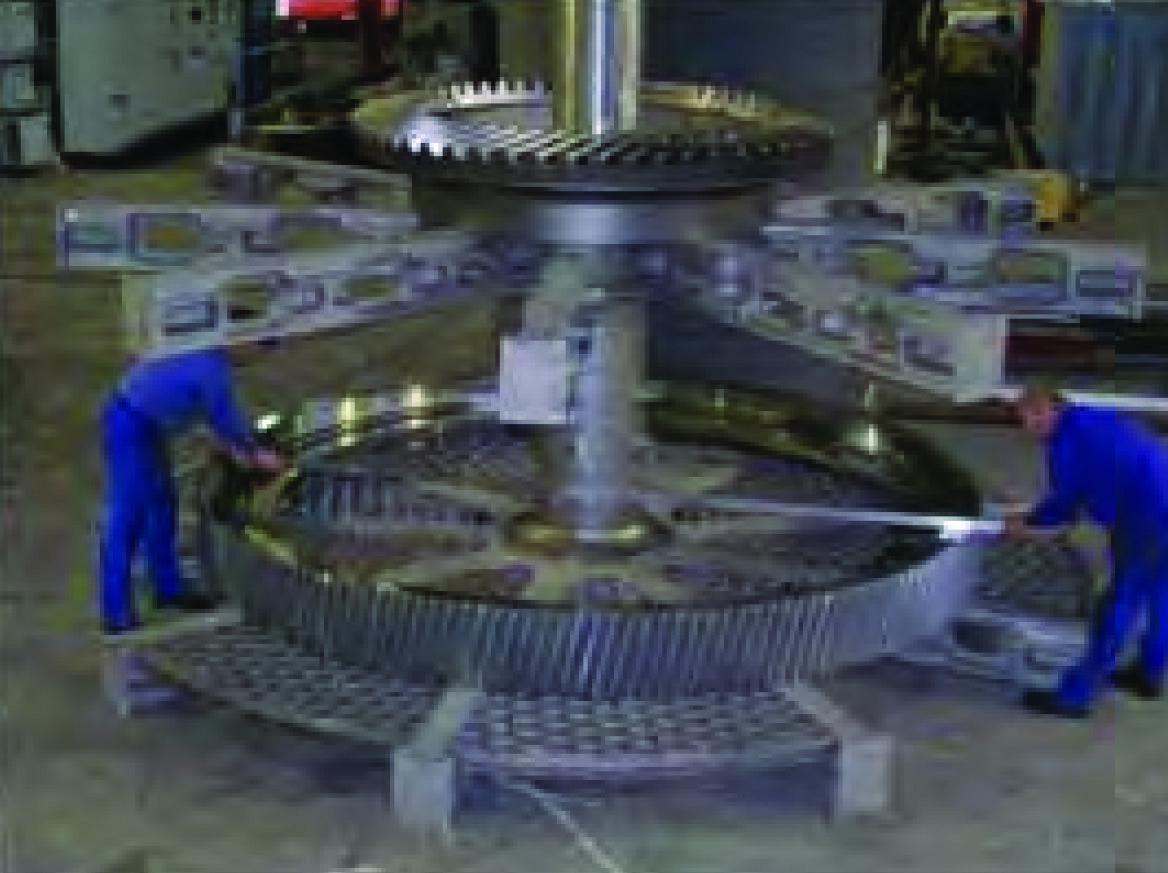

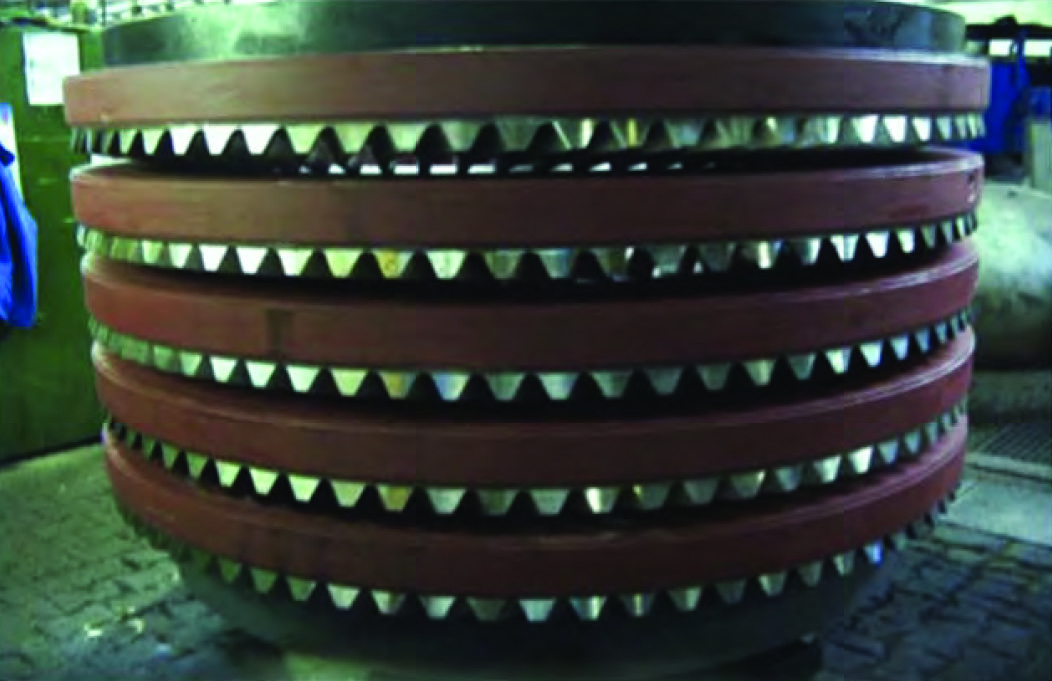
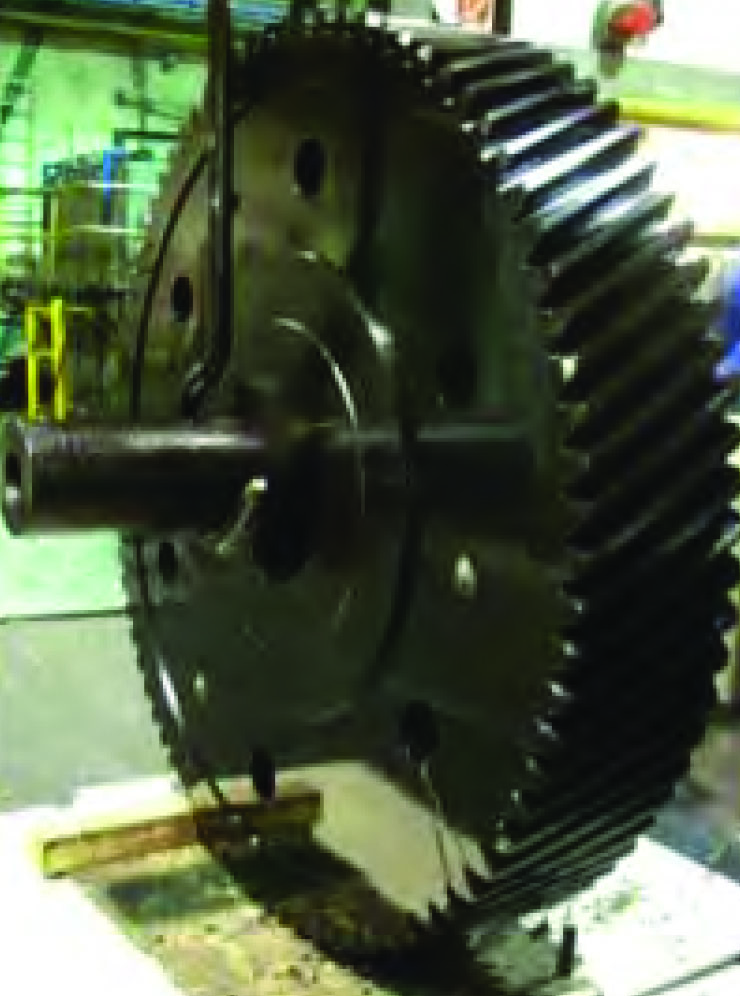

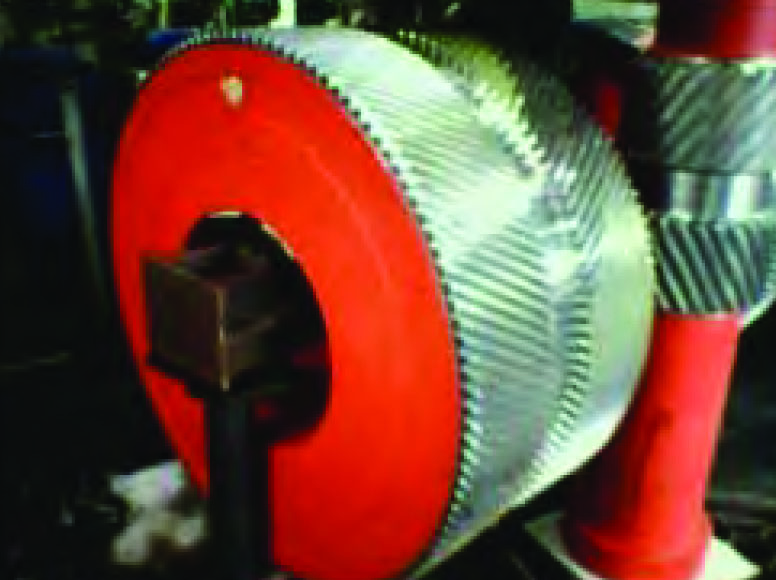
Practical Experience of the Shop Manager (Case Studies)
For the successful low distortion heat treatment, it takes a lot of experience, and here are a few practical experiences of the REESE shop manager Klaus Hölken. Many German customers have expressed that Reese treated gears have better dimensional stability compared to competitors, and captive heat treating facilities.
Process Endorsement
- A Canadian gear manufacturer contacted Reese about gear rings with up to 30 mm distortions, mainly in the form of ovality. The question was posed, “What could be done to improve such deviations?” The gear heat treatment was completed with deviations below 0.30 mm with respect to wobble and ovality.
- A French gear manufacturer only gave us pinion shafts for treating in the early stages. The first wheels were not very good, but still at a good quality. The growth scattered and tapering, ovality, and runout (out of flatness) were not as good compared to gears for other customers. The shop manager gave extensive material recommendations and advice for the pre-treatment and also stated that Reese would harden wheels of around 2 m in diameter vertically—not horizontally. The customer showed a mass of gears the competition had previously hardened with 5 mm tapering. Although the optimization possibilities of the material were not fully implemented yet, Reese achieved an average runout, tapering and ovality of <0.5 mm with a maximum chance of values of 0.7 mm. The ovality and tapering of up to 0.7 mm was caused due to the remaining bandings in the material. Uncontrolled growth is reduced by narrowing the hardenability (alloy) scatter bands and is now available in narrower limits.
- The gear wheels of a customer in Spain have come out very well, according to the measurement capabilities.
- Another long-time customer in France had bad experience with Reese competitors. There was dissatisfaction with the core strengths of their case-hardening process. The customer has now completely committed to the Reese program. Some parts are among the relatively smaller group of components, but are still very distortion sensitive.
Process Outcomes
Vertical hardening that has been implemented on a large scale with specially designed charging methods is much better than the horizontal versions in many cases. Also, wheels that can only be treated horizontally because of the geometry, can now be hardened much better because of special adjustable supports used. Solid wheels grow in general the larger the tooth face width. If the tooth width is relatively small in relation to the diameter the wheels grow by about 1-1.5 mm. If the tooth width is larger, growth will rise to 2-2.5 mm. For very large tooth widths, such as gears for turbo transmissions, growth trends to 3-3.5 mm and more can be reported.
Welding constructions generally keep their dimension or grow up to one millimeter. Materials from 16/20MnCr5, (solid wheels and weldments) without banding or largely free of banding, grow only slightly by <1mm. In the presence of banding or even mixed grain sizes, growing can occur as well as contraction (directed growth). A safe prediction is not possible, and often results in scrap. The directed growth may be a result of a smaller outer diameter, a smaller bore or greater tooth widths.
When using a 18CrNiMo7-6 in the tempered state (thus largely free from banding and mixed grain), in the version as HH-quality and with a vertical charging, Reese has experienced runout flatness, roundness and conicity/tapering of well below 1 mm. Banding in the material can lead to ovality and tapering.
An angular deviation of the toothing is also recorded with banding structure and with a lack of supporting material, or if the surfaces are too small below the teeth. If the tooth width is very small compared to diameter, angular deviations occur in the teeth. Also, the bore diameter and the hub have an influence on strength of the angular deviation of the toothing. Reliable predictions of dimensional developments can be made very accurately when: the material is good and ordered under reproducible conditions; it is measured before and after heat treatment; and hardening of a good recurring alike is ensured. High quality and exceptional charging methods along with heat treatment uniformity helps achieve optimal quality of production and material ordering.
Simulations
Computer programs are available to simulate possible results, as seen in Figure 25 and Figure 26, based on different charging techniques before treatment. An example of a simulation of the hardening distortion of a spur gear with horizontal charging can be seen in:
- Conicity up to 3.5 mm
- Axial run-out up to 3.8 mm
- Damages due to supporting points

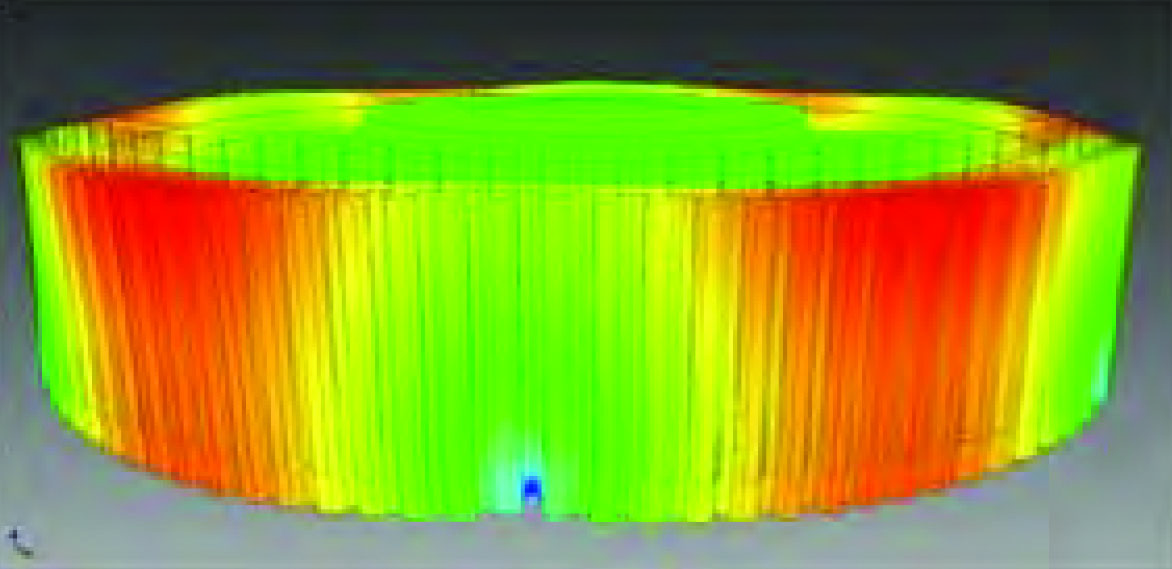
Statistical Evaluations
After the heat treatment, regular sampling evaluations are performed. In Figure 27 and Figure 28, the same gear wheel was evaluated after both vertical and horizontal loading. By vertical batching of gear wheels the axial runout and conicity, induced by heat treatment, can virtually be eliminated. Also ovality can be minimized with special measures. From the statistical tests and simulations, typologies (Table 4) and trend graphs for dimensional changes are derived. Figure 29 and Figure 30 both exemplify trend graphs for dimensional changes.
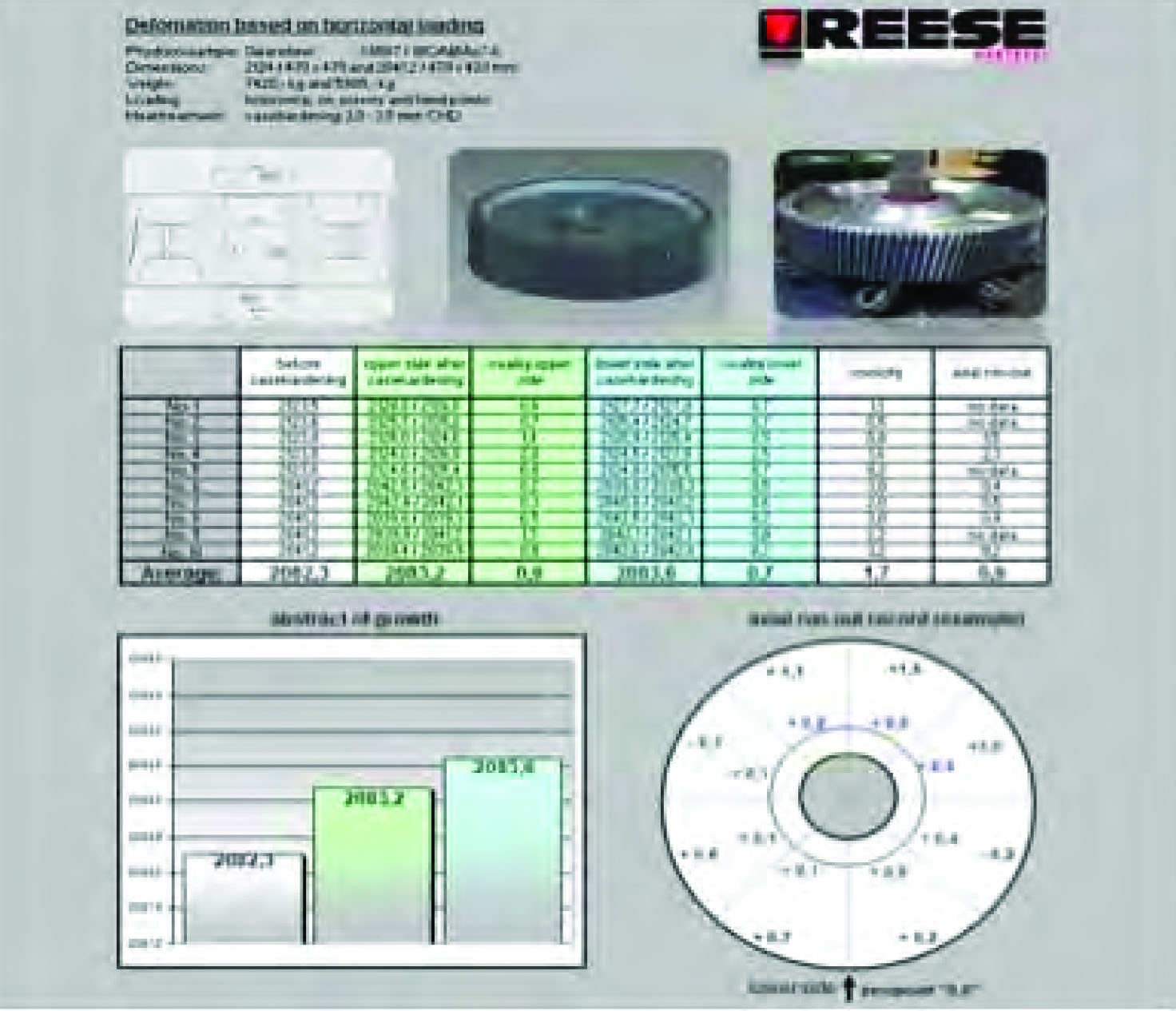

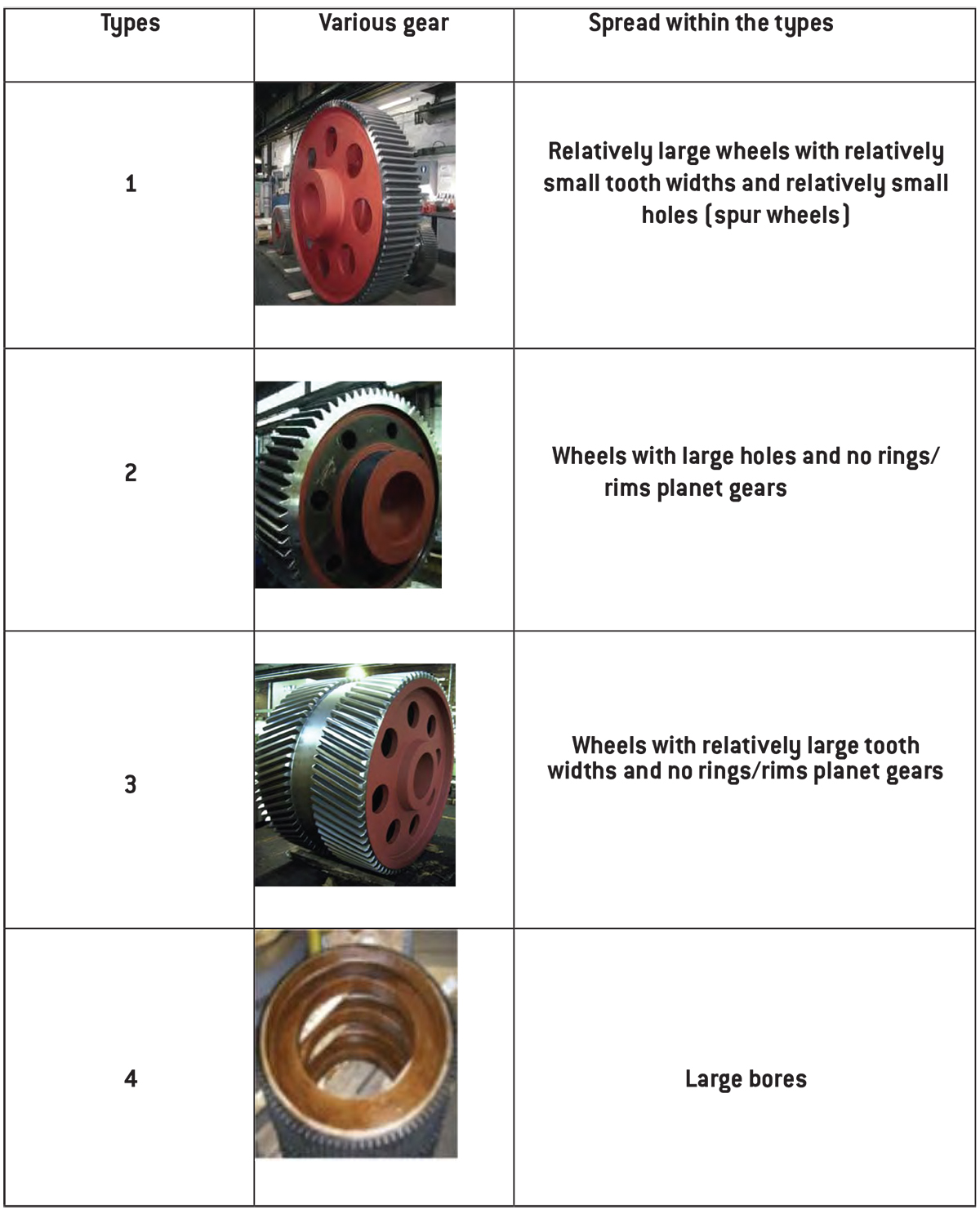
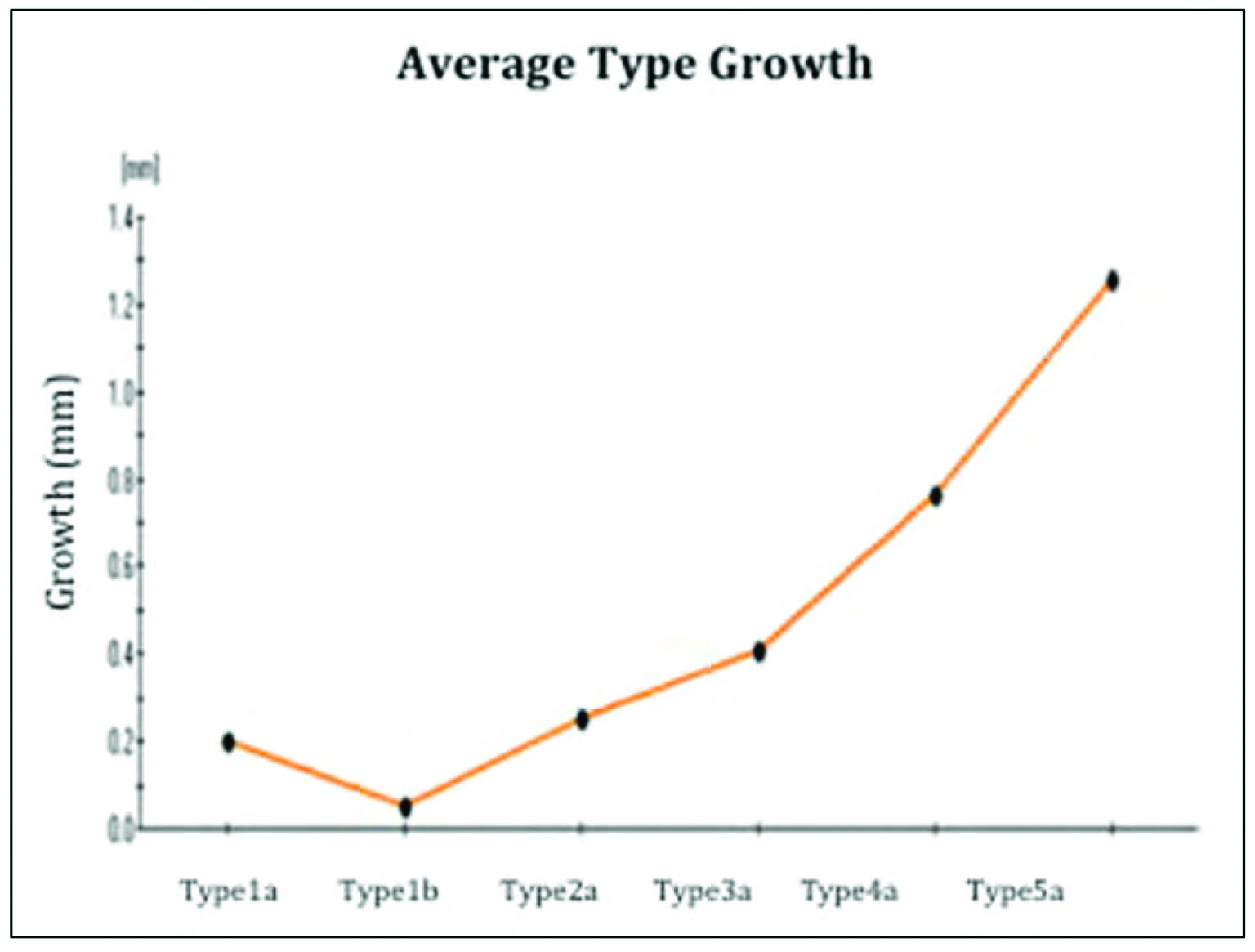

Conclusions
Because of the highest load capacity, case hardening has proven to be first choice for the treatment of large transmission components. Steel selection, conditioning heat treatment, hardenability, weight, dimensional changes and distortion are the main aspects that must be met before case hardening results are optimal. If these factors are perfectly harmonized the existing technical limits can be exceeded, which opens up entirely new possibilities for the gear manufacturers.
In this process, I particularly came to a decisive and crucial factor: the distortion during the hardening process. The hardening distortion has proven to be the crucial problem in the past. Reese Bochum has created the conditions to master this crucial problem of hardening distortion by the technique of vertical hardening. In addition, very low hardening distortion results can be achieved through special techniques, even with horizontal charging of gear rims and bevel gears.
References
- Benkowsky, G. Induktionserwärmung (213-227). VEB Verlag Technik Berlin, Berlin (1980).
- Weiß, T. “Zum Festigkeits- und Verzugverhalten von randschichtgehärteten Zahnrädern.” Dissertation Technische Universität München (1983).
- Specht, F. “Induction Heat Treating Off-Highway Components.” In Heat Treating Progress (22-25), Volume 3, Number 2, March 2003.
- Marquis, F. “Traitment thermique par induction, suivi de qualité en temps reel.” Traitment Thermique (23-27) 368, Janv.-Fév. (2006).
- Schwenk, W., Peter, H.J. “Anwendungen des Zweifrequenz-Simultan-Verfahrens zum induktiven Randschichthärten.” Elektrowärme Internationl, Heft 1 (13-18), März 2002 (2002).
- Möckel, F., Werner, J. “Praktische Erfahrungen beim Induktivhärten schrägverzahnter Bauteile.” Neue Hütte, 26 (391-92) (1981).