Editor’s note » This is part two of a three-part series on carburized steel mechanical properties.
In part one, it was determined the carburized case was not as strong as the hardness would predict. How are carburized gears able to function as well as they do since in bending, the applied stress is greatest at the surface? Most of the information in this part and part three has been in the public domain since 1988, but it is timeless and will help to understand this question.
In the mid-1980s, there was an issue with low-impact life on a light truck rear axle during vehicle durability testing. The differential gears were failing after 3-5 cycles of a vehicle clutch dump test (rev engine up and slide foot off clutch). This was back at a time when the teeth on differential gears were still machined rather than net formed. The Revacycle machined gears were made from 8615 carburized steel with a case depth of about 0.89 mm and a core hardness of about 30-35 HRC. The failure mode was bending overload at the tooth root radius of the differential pinion.
Studying grades of carburizing steel
A study was initiated to look at different grades of carburizing steel and the effects of tempering temperature [1]. Unnotched Charpy bars were used because of a concern the properties of notched bars might be too low to show any meaningful difference. The data for this study is shown in Table 1. Tempering at elevated temperatures was evaluated because it was being used to increase impact strength in some drag racing applications where gears were changed out every weekend and longevity was not an issue.
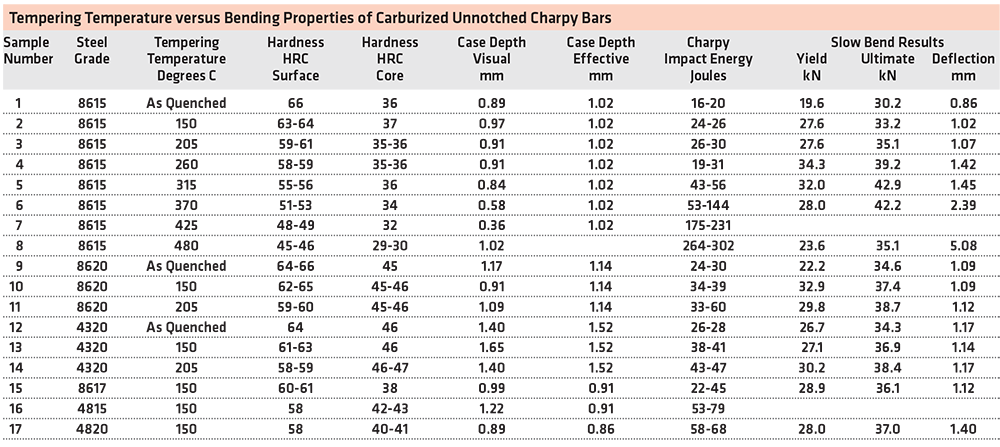
In bending, the stress should be maximum at the surface and zero at the center of the cross section. Based on hardness, carburizing is ideal because it places the high hardness and strength at the surface where it is needed most. As the tempering temperature increases, the surface is softened, which should decrease the strength. However, if we look at Figure 1, we can see the ultimate strength actually increases with increasing tempering temperature and reaches a maximum at about 316°C where the surface hardness has decreased from 66 HRC to about 52 HRC.
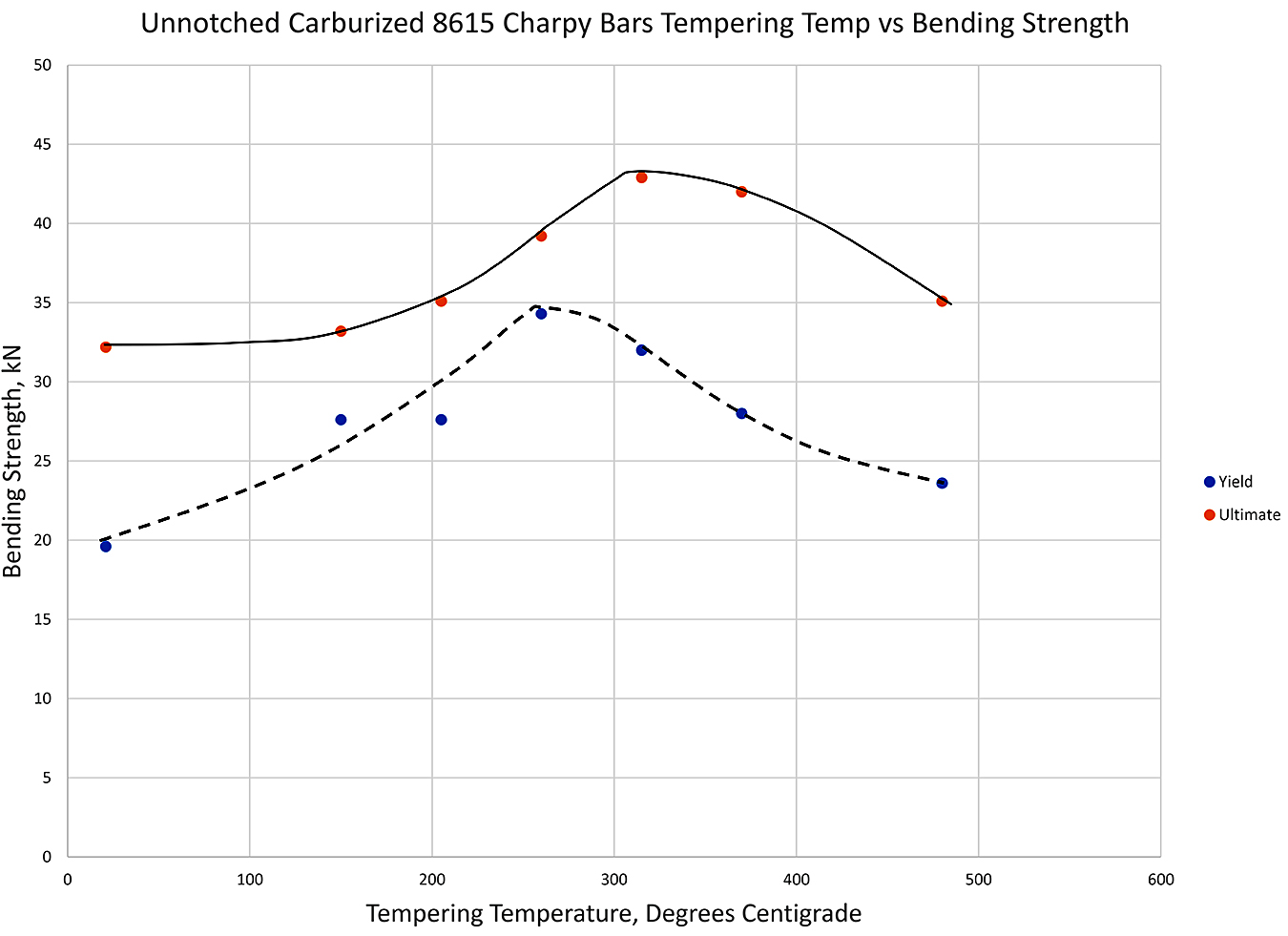
Likewise, the yield strength as determined by the Johnson Elastic Limit or JEL (50% change in slope) reaches a maximum at a tempering temperature of 260°C where the surface hardness has decreased to 59 HRC. This indicates the carburized case is not as strong as the hardness indicates. As we saw in part 1, it is suffering from a form of embrittlement called quench embrittlement [2]. The carburized case is not able to reach its full potential strength based on hardness because it fails in the elastic region before any significant plasticity occurs. This is not an anomaly, but rather it is normal for carburized components. It is a prime example of a brittle material that has been around for a long time and still functions very well in demanding applications.
Embrittlement issue
Tempering at an elevated temperature will certainly address the embrittlement issue to a degree; however, wear and fatigue life will then become major concerns.
Quench embrittlement does not normally occur with hardened lower carbon steels. However, a similar issue due to temper embrittlement was documented on a medium carbon 1038 induction hardened shaft tempered on the same scanning equipment used for hardening [3]. Elevated temperature tempering after the fact had the same effect of increasing the bending strength with decreasing hardness. With furnace tempered non-embrittled shafts, the bending strength and ductility remained constant up through 300°C. The embrittled shafts exhibited an intergranular fracture at the origin, while the normal furnace tempered shafts also exhibited intergranular fracture but with a small amount of dimple rupture fracture.
Increasing impact strength
The Unnotched Charpy bar data in Table 1 indicated 4320 steel should be a better choice for increasing the impact strength of the differential gear compared to 8615. The 4320 tempered at 150°C showed an increase in ultimate strength from 33.2 kN to 36.9 kN, and the impact energy increased from 24-26 J to 38-41 J. The test bar data also showed the increase in core hardness with 4320 should not be an issue. Figure 2 shows increased core should increase bending strength. Gears were made with 4320 steel and the truck impact test was rerun, and the life decreased to only 1-2 cycles. The test bar data did not appear to agree with the actual parts.

The main difference between the test bars and actual parts was the parts had a root radius to increase stress while the test bars did not. As a result, a new series of one-inch-round test bars with six inches between supports was designed. There was a smooth test bar without a radius, which is shown in Figure 3 and shouldered bar with a radius shown in Figure 4.
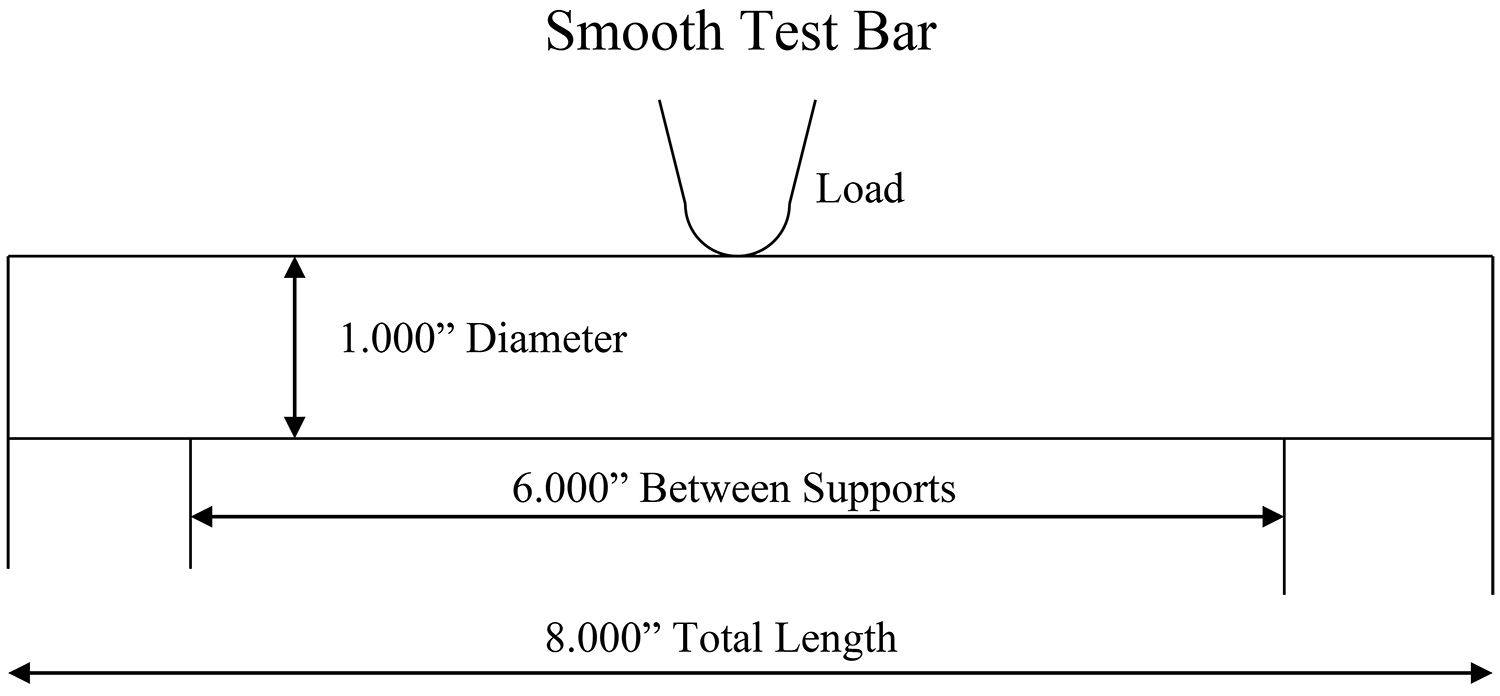

Evaluating core hardness
To evaluate the effect of core hardness, the carbon content and resulting hardenability was changed within the 8600 series low nickel family (8615, 8620, 8625, 8630, 8640), and the hardenability was changed using alloy content within the medium nickel family (4620 and 4320). These bars were tested under slow bend, drop tower impact, and fatigue conditions. The slow bend results for the smooth bar are shown in Figure 5 [4].]

The smooth bar results were similar to the unnotched Charpy data in that higher core hardness appears to provide higher strength. The spread between bending yield, as determined by the JEL, and bending ultimate decreases as the core hardness increases. The medium nickel steels 4320 and 4620 and the high nickel steel 9310 appear to provide a significant improvement over 8600 series low nickel steels. Once again, this is not in agreement with the actual differential gears.
The shouldered test bar results are shown in Figure 6 [5]. With the shouldered bar, there is an increase in bending yield and ultimate strength with increasing core hardness but only to about 30 HRC where the yield remains constant and the ultimate strength decreases to meet the yield strength at about 40 HRC. This potentially explains why the 4320 differential gears with a core hardness of 42-45 HRC performed worse than the 8615 gears with a core hardness of 30-35 HRC. The data does indicate the rules that govern carburized parts in bending are significantly different depending on whether a radius or stress concentration is present or not. A smooth bar without a stress concentration will not provide the correct information for a gear tooth. Higher core hardness is beneficial for the smooth bar, and it is detrimental for the shouldered bar. With the shouldered test bar, the optimum core hardness for low nickel steels and possibly medium nickel steels is about 30-35 HRC. It provides the highest yield and ultimate strength. Below this hardness, the yield and ultimate strength will decrease, and above it, the ultimate strength will decrease to meet the yield strength.

Impact strength data
Figure 7 shows the shouldered bar impact strength data from the instrumented drop tower test [5]. Like the slow bend test, the strength increases with increasing core hardness but only to a point. The yield and ultimate strength also become the same at about 40 HRC. Unlike the slow bend data, 4320 does show an improvement over the 8615 steel. However, the 4320 core hardness is considerably lower than the actual gear due to the one-inch cross section of the test bar. Another notable difference from the slow bend test is the bending strength is higher under impact conditions.
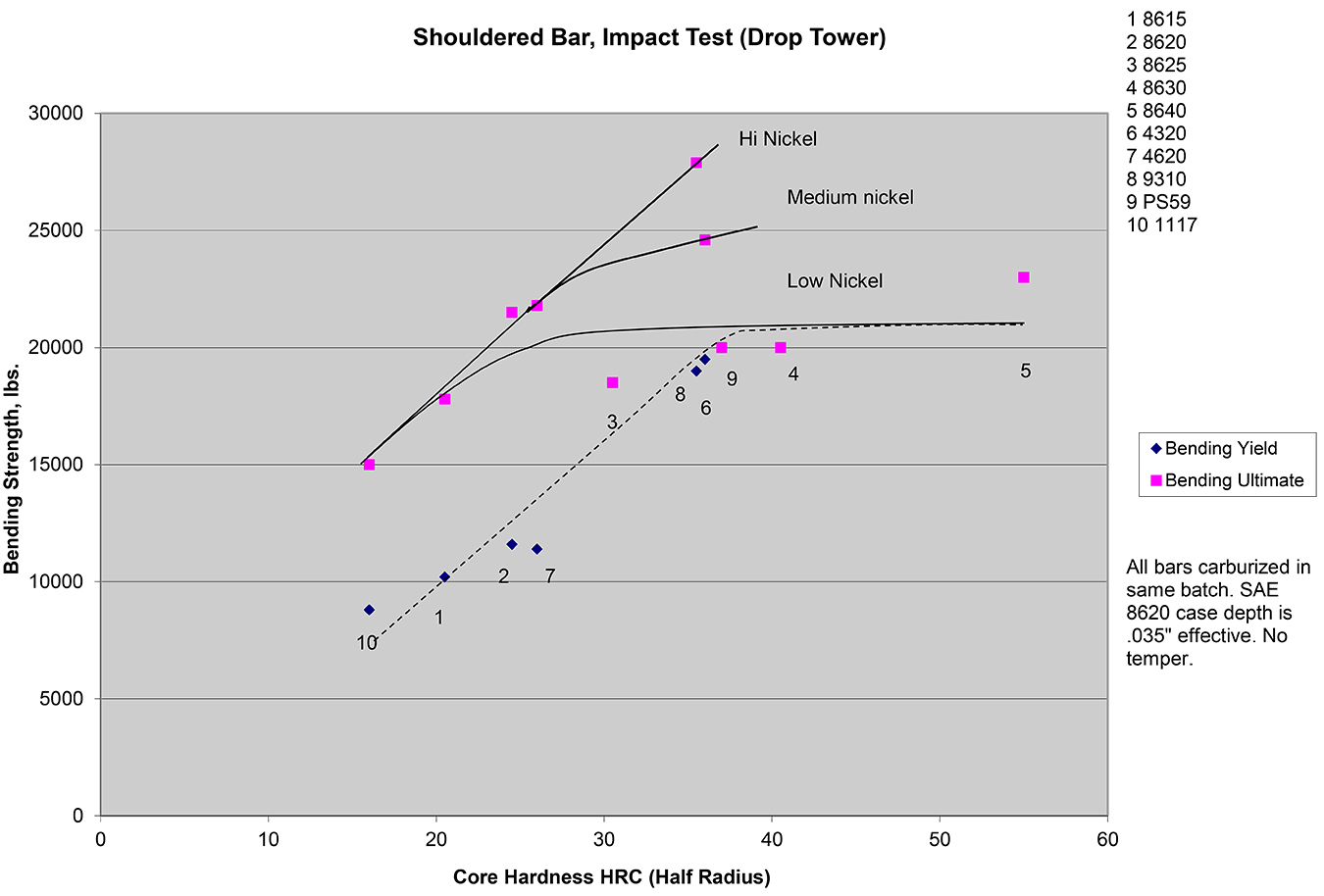
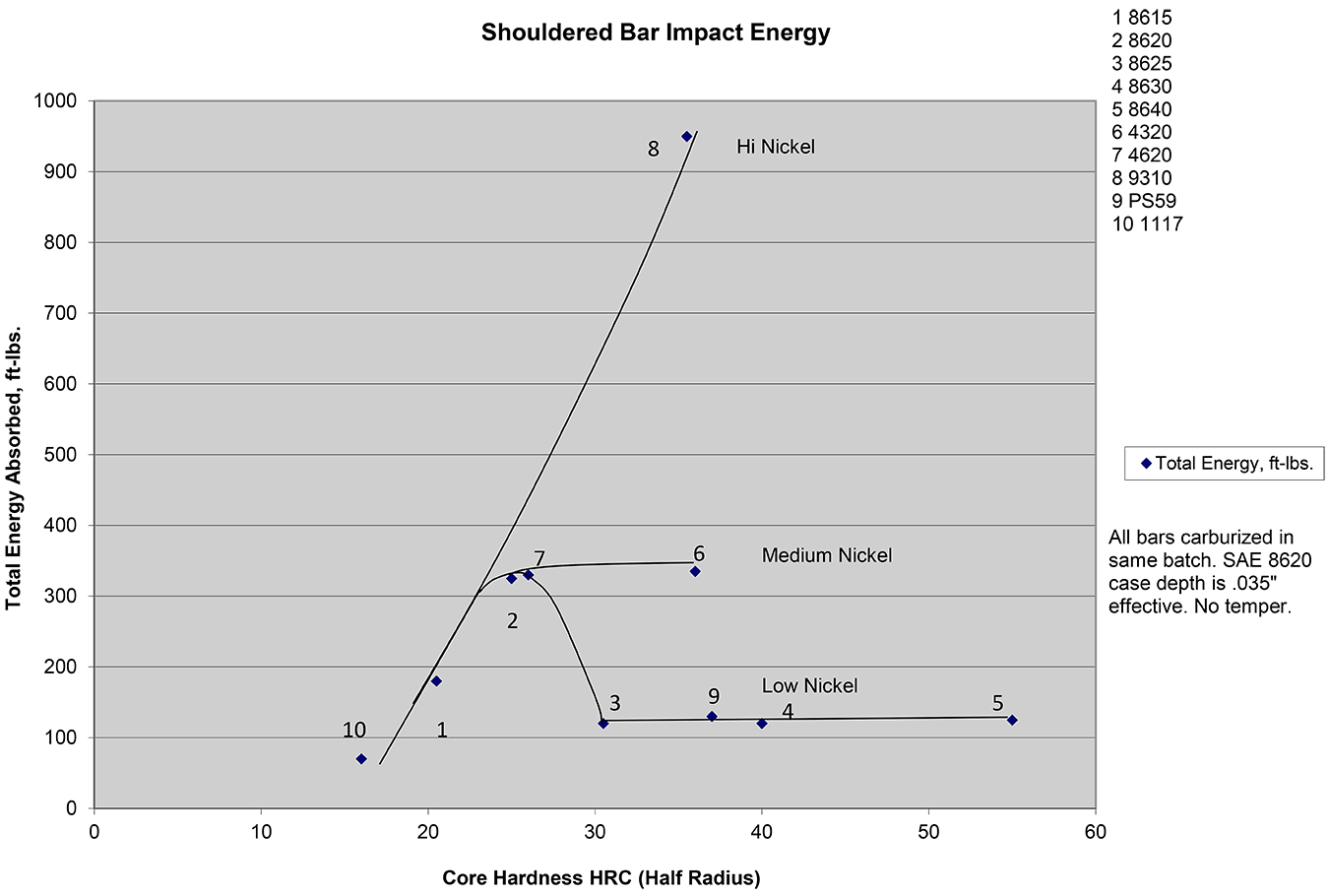
Figure 8 shows the shouldered bar absorbed impact energy from the drop tower test [5]. The energy initially increases with increasing core hardness to about 350 ft-lbs at 25 HRC. The energy then decreases for the low nickel steels to 120 ft-lbs at 30 HRC remains level above that. The energy for the medium nickel 4620 and 4320 steels remains level at 350 ft-lbs at 25-35 HRC. The high nickel 9310 steel has the highest energy at about 950 ft-lbs at 35 HRC. This data also indicates there should be an improvement in impact life for the 4320 steel over the 8615 steel. However, due to the test bars cross section, the core hardness values are lower than the actual gears.
In part three, a smaller test bar that duplicates the core hardness of the actual gear will be discussed, as well as the effect of case depth.
References
- G. Fett, “Tempering of Carburized Parts,” Metal Progress, September 1982, Pages 53-55.
- J. Wong, D. Matlock, G. Krauss, Effects of Induction Tempering on Microstructure, Properties and Fracture of Hardened Carbon Steels,” Proceedings of 43rd Mechanical Working and Steel Processing Conference, ISS, October 2001.
- V. Rudnev, G. Fett, S.L. Semiatin, Tempering of Induction Hardened Steels, ASM Handbook Volume 4C Induction Heating and Heat Treatment, 2014, Pages 151-152.
- Unpublished work done by G. Fett Dana Corp., Circa 1985-1988.
- G. Fett, “Bending Properties of Carburized Steels,” Advanced Materials and Processes Incorporating Metal Progress, April 1988, Pages 43-45.