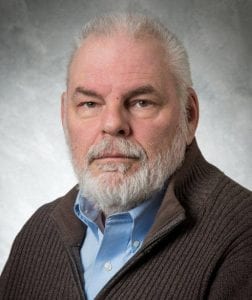
In this column, we will discuss the effect of boron additions to steel and its influence on properties.
Boron, B, is an element with an atomic number of 5, adjacent to Carbon, C, on the periodic table. Boron has an atomic weight of 10.81 g/cm3 and is a solid at standard temperature and pressure. The typical crystal structure is rhombohedral and was discovered by Joseph Gay-Lussac and Louis Jacques Thenard in 1808. Elemental boron is difficult to produce because it readily oxidizes.
Boron is most found as borax (Na2B4O5(OH)4·8H2O) or boric acid. There are approximately 100 borate minerals known [1]. Boron is not found in uncombined form on Earth but is occasionally found as a metalloid in meteoroids.
Boron is used in many different applications. When woven as a fiber, it is used in aerospace to create lightweight composite structures. These woven fibers have also been used to create high-end consumer shafting, such as lightweight fishing rods and golf club shafting.
Borosilicate glass is another use of boron. This type of glass has a low coefficient of expansion and is used for telescope lenses and mirrors. It has good resistance to thermal shock and is used for labware and cookware. In the United States, it is trademarked as PYREX™.
Application in steel
Boron is commonly added to steels to increase hardenability. Boron is added at a very low concentration of 0.0015 to 0.003 percent to increase the hardenability of low carbon steel. Boron is added to molten steel as a ferro-boron which can contain as much as 20 percent boron. The ferro-boron is typically added to the ladle after all the deoxidizers and other alloying elements have been added because boron reacts strongly with oxygen, carbon, and nitrogen.
Boron reacts with oxygen to form B2O3, and reacts with carbon to form a boron cementite, Fe3(CB). Or an iron boron carbide, Fe23(CB)6. Boron reacts with nitrogen to form boron nitride (BN). To prevent loss of effectiveness of boron due to loss to oxidation, the use of higher aluminum or titanium content is usually used during melting.
During rolling and hot working, boron steels are like carbon steels. Carbon and boron have similar diffusion coefficients, so loss at the surface can occur at high temperatures and oxidizing atmospheres. Use of low nitrogen atmospheres is recommended to prevent the formation of boron nitride (BN).
If the boron content exceeds approximately 0.007 percent, hot shortness during rolling or forging can occur. Poor impact properties also occur at this higher boron content. The hot-shortness and poor impact properties are due to a low-temperature iron-carbon-boron eutectoid (Fe/Fe3C/Fe2B) that forms when the boron content exceed 0.007 percent [2]. Boron steel usually has a “B” in its description, for instance, an SAE 1035 steel that has had boron added to increase hardenability is designated as a SAE 10B35 steel.
Boron can have different locations in the iron lattice. Like carbon, boron has been found to occupy interstitial sites [3]. This was based on X-ray diffraction measurements of the austenite lattice parameter [4]. As temperature is decreased, the austenite lattice parameter of boron-containing steel was increased. This suggests that the boron migrates from the interstitial sites to the grain boundaries.
The biggest advantage to adding boron to a steel is the increase in hardenability that occurs. Even very small amounts of boron can drastically increase the hardenability of even low carbon steels. Boron tends to segregate to austenite grain boundaries, which then retards the austenite to ferrite, pearlite, and bainite transformation [5]. Because of the segregation of boron to the grain boundaries, embrittlement can occur. A reduction in toughness and ductility can also occur [6].
The increase in hardenability is illustrated by Figure 1. In this figure, the Jominy curve of a simple SAE 1020 steel (0.2 %C, 0.25% Si, 0.5% Mn) is compared to the calculated Jominy curve of an identical steel with 0.003 percent boron added.

As can be seen from Figure 1, the very low hardenability of SAE 1020 is increased substantially by the addition of 0.003 percent boron. The depth of hardening is increased approximately from J = 1/16” to J = 4/16”. This is a large improvement that can be readily achieved.
Looking at more detail into the microstructure make-up (Figure 2), as a function of quench rate (distance from the quenched end), it can immediately be seen that the boron-containing steel at the quenched end is entirely composed of martensite, while the non-boron steel has a reduced martensite content. In the boron-containing steel, the microstructure is predominantly martensite out to approximately 0.25”, while at 0.25” from the quenched end, the non-boron sample contains only bainite and ferrite.

Boron is unique in that it has no effect on the martensite start (Ms) transformation temperatures. During tempering, an increase in embrittlement can occur, and different temperatures are required for tempering to achieve the same hardness [6].
Conclusions
In this article, we have shown the beneficial increase in hardenability by small additions of boron. Boron steels are used extensively as an inexpensive replacement for more highly alloyed steels.
Should there be any questions or comments regarding this article, or suggestions for other articles, please contact the editor or myself.
References
- C. Klein and B. Dutrow, Manual of Mineral Science, New York: John Wiley & Sons, 2007.
- S. K. Sarna, “ISPAT Guru,” 21 11 2014. [Online]. Available: https://www.ispatguru.com/boron-in-steels/.
- J. E. Morral and T. B. Comeron, “Boron Hardenability Mechanisms in Steel,” TMS/AIME, p. September, 1979.
- R. M. Goldhoff and J. W. Spretnak, “Distribution of boron in gamma iron grains,” JOM, vol. 9, pp. 1278-1283, 1957.
- Key to Metals AG, “Total Materia – Boron in Steel: Part Two,” November 2007. [Online]. Available: https://www.totalmateria.com/page.aspx?ID=CheckArticle&site=kts&NM=214.
- B. M. Kapadia, “Effect of Boron Additions on Toughness of Heat Treated Low-Alloy Steels,” Journal of Heat Treatment, vol. 5, no. 1, pp. 41-53, 1987.