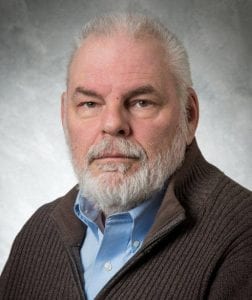
When slow quenching 2024 aluminum, this alloy is susceptible to intergranular corrosion. Two common quenchants, water and AMS 3025 PAG type quenchants, are compared.
Introduction
Heat treating aluminum requires attention to all process parameters to ensure that properties are achieved, without excess distortion or susceptibility to corrosion. Polymer quenchants such as those described by AMS 3025 [1] were developed to accomplish the goal of achieving properties, reducing distortion and residual stresses, while achieving similar corrosion susceptibility as those parts quenched in water. The concentrations of Type I and Type II quenchants, specified by AMS 2770 [2], used for quenching of aluminum, are based on the “zero-delta” concept. In other words, properties of parts quenched in AMS 3025 quenchants will have identical properties as those quenched in water.
Schematically, the heat-treatment process is shown in Figure 1. Of each of these processes, quenching is the most important step to achieving mechanical properties, low residual stresses, and reducing corrosion susceptibility.

As a part is quenched, the solute is held in supersaturation until the part is cooled. Properly quenched, all the solutes will remain in supersaturated solid solution until natural aging commences once the part has cooled to room temperature. This requires a fast quench. If the part is quenched at a rate less than the perfect rate, heterogeneous precipitation will occur, with equilibrium precipitates forming at the grain boundaries. The rate at which precipitation occurs is dependent on the degree of supersaturation, and the diffusion rate. But the diffusion rate increases as a function of temperature. The diffusion rate is greatest at elevated temperature. When either the supersaturation or the diffusion rate is low, the precipitation rate is low. At intermediate temperatures, the amount of supersaturation is relatively high, as is the diffusion rate. Therefore, the heterogeneous precipitation rate is the greatest at intermediate temperatures. The amount of time spent in this critical temperature range is governed by the quench rate.
The amount of precipitation occurring during quenching reduces the amount of subsequent hardening possible. This is because as solute is precipitated from solution during quenching, it is unavailable for any further precipitation reactions. This results in lower tensile strength, yield strength, ductility, and increased corrosion susceptibility.
In aluminum alloy 2024, the inter-granular corrosion susceptibility is driven by the presence of Al2Cu (θΘ) and Al2CuMg equilibrium precipitates at the grain boundaries. The region is surrounded by a precipitate free zone (PFZ) that is essentially pure aluminum and is depleted of solute. This is shown in Figure 2. The difference in electrochemical corrosion potential between the grain boundary and the PFZ creates a galvanic cell, and intergranular corrosion follows this path. Specifically, the copper rich precipitates and grain boundary regions act as local cathodes, which drive anodic dissolution of the precipitate-free zones.
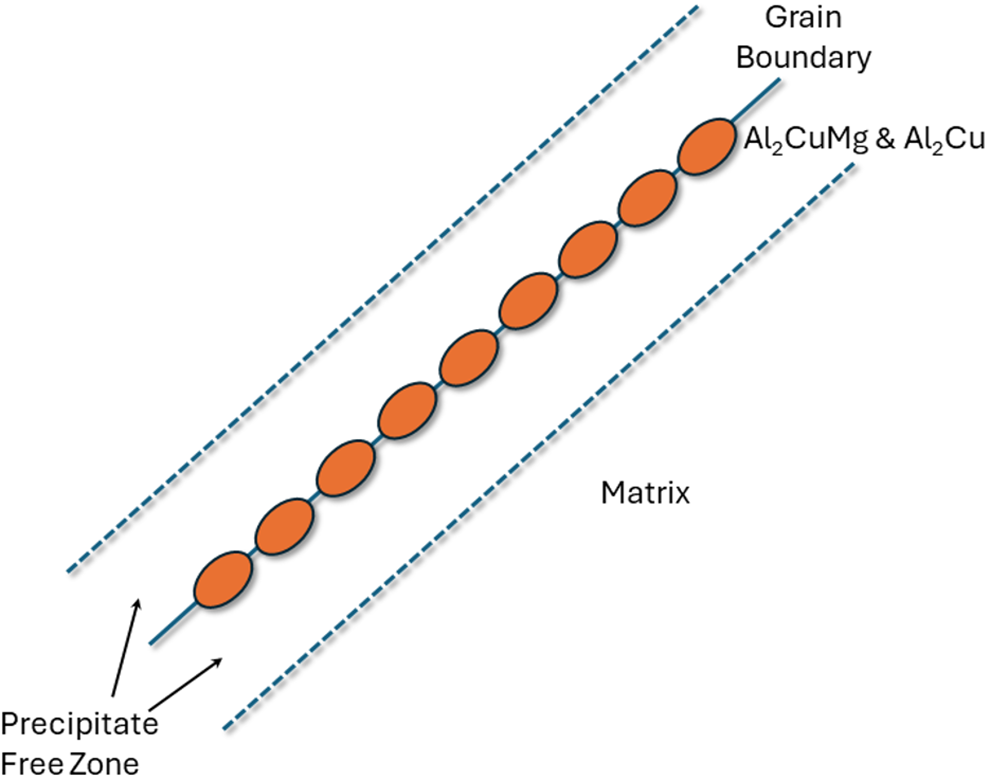
Procedure and results
Sixteen 150 x 225 mm panels of 1.8 mm thick 2024 aluminum sheet, consisting of four different heat lots, were solution heat treated at 493°C (935°F) and quenched in either water or 28% AMS 3025 Type I PAG quenchant. Panels were then naturally aged to the -T4 temper for 96 hours. Two panels from each heat lot were quenched in water, and two panels from each heat lot were quenched in 28% AMS 3025 Type I PAG quenchant. The concentration of 28% is the maximum percentage of AMS 3025 [2] permitted for this alloy and thickness per AMS 2770 [3]. The panels were then corrosion tested per ASTM G110 [4], except the immersion period was seven hours. Three 25 x 25 mm samples were sectioned from each panel and examined at 100X through 500X before and after etching with Keller’s Etch [5]. The depth of intergranular corrosion was measured and compared to the water quenched panels. The results are tabulated in Table 1.
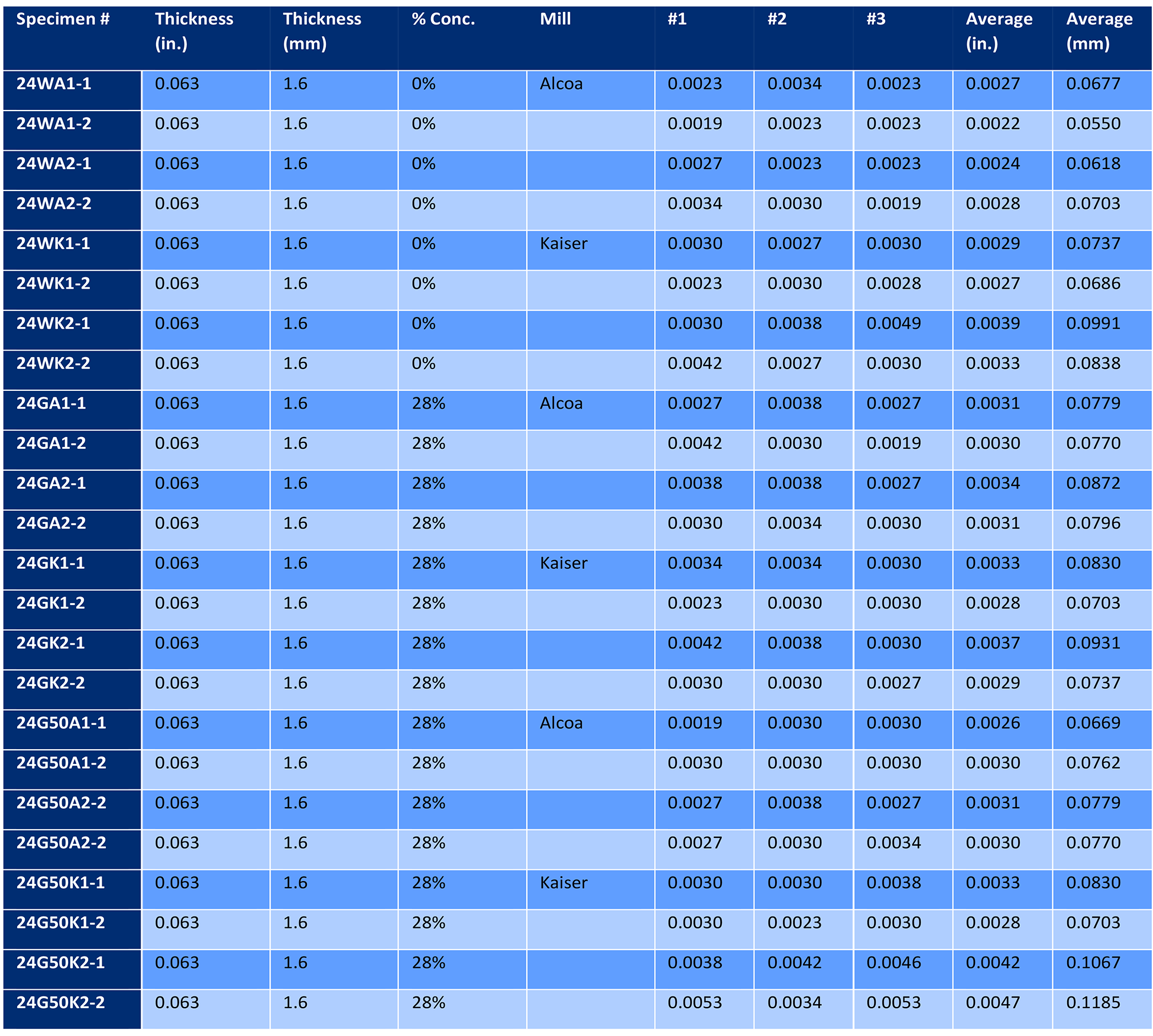
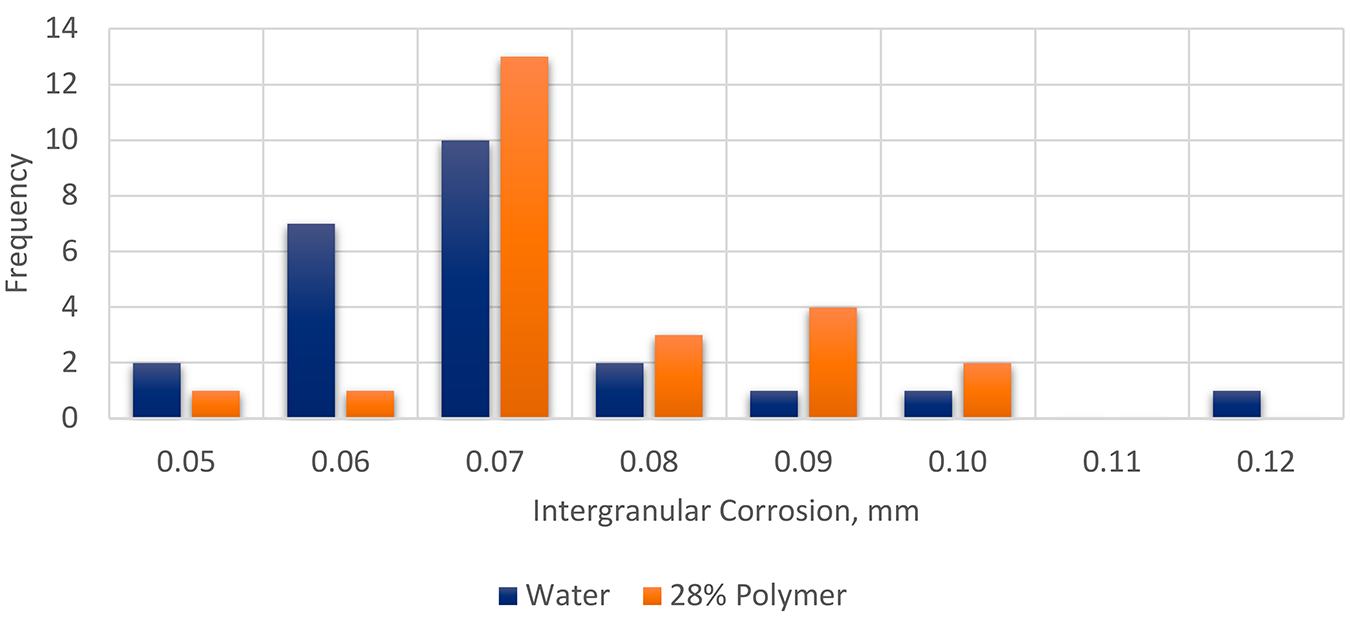
A comparison of the depth of intergranular corrosion (Figure 3) shows that the recommended maximum concentration for polymer quenchant (28%) produced similar depths of penetration of intergranular corrosion as those that are water quenched. Further, there was less overall scatter in the results of the polymer quenched panels.
Twenty-four additional panels, eight from a single heat of 3.0 mm sheet, eight from one heat of 5.0 mm sheet, and eight from a single heat of 8.0 mm plate, were also solution heated-treated, quenched, and naturally aged. Two panels of each thickness were quenched in cold water. The balance of panels in each thickness was quenched in three different concentrations of Aqua-Quench™ 260 (an AMS 3025 Type I quenchant). These concentrations were 15%, 28%, and 45% respectively. Three 25 x 25 mm samples were sectioned from each panel and examined at 100X through 500X before and after etching with Keller’s Etch [5]. The depth of intergranular corrosion was measured and compared to the water-quenched panels. The results are tabulated in Table 2. The resultant data is shown in Figure 4.
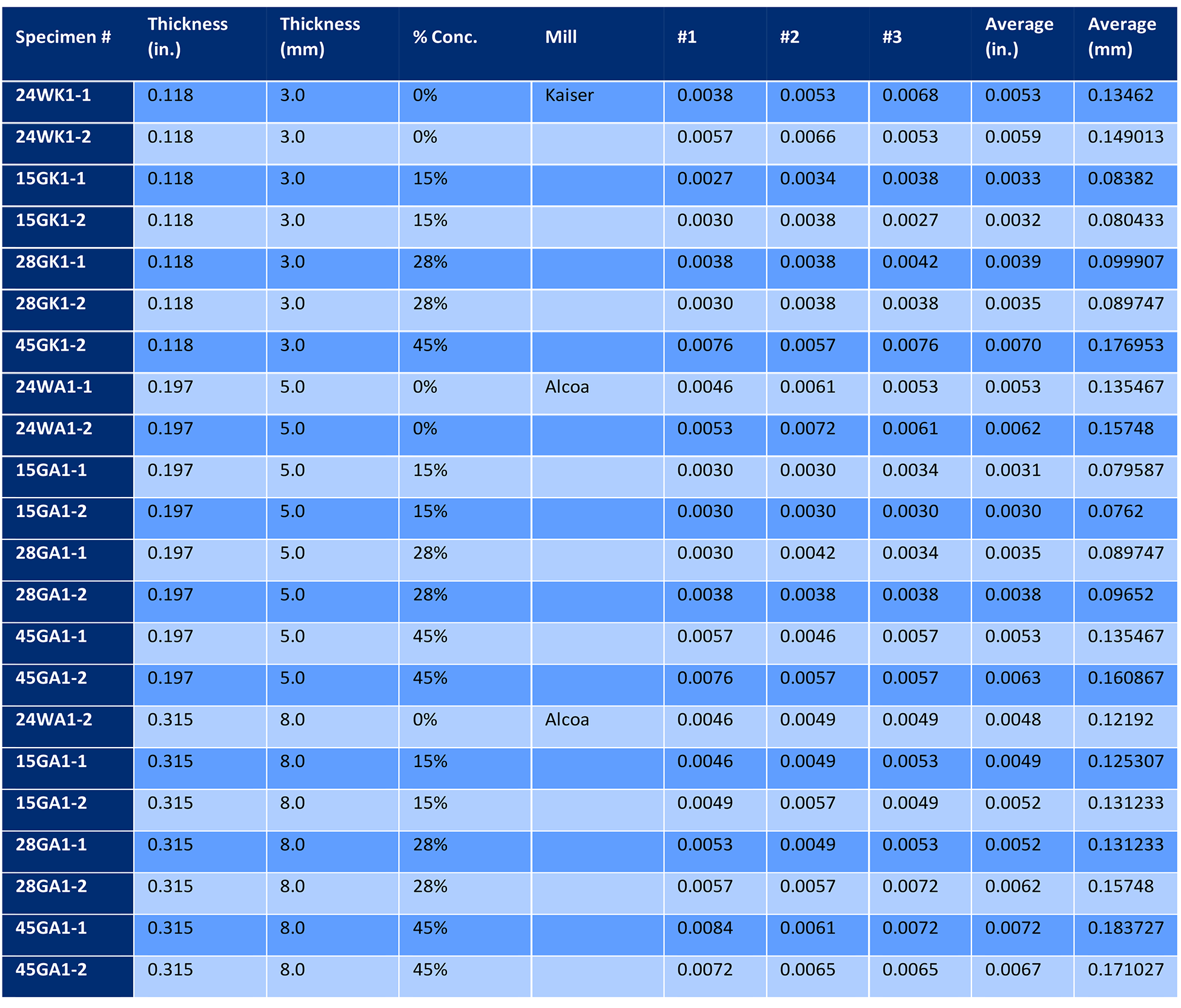
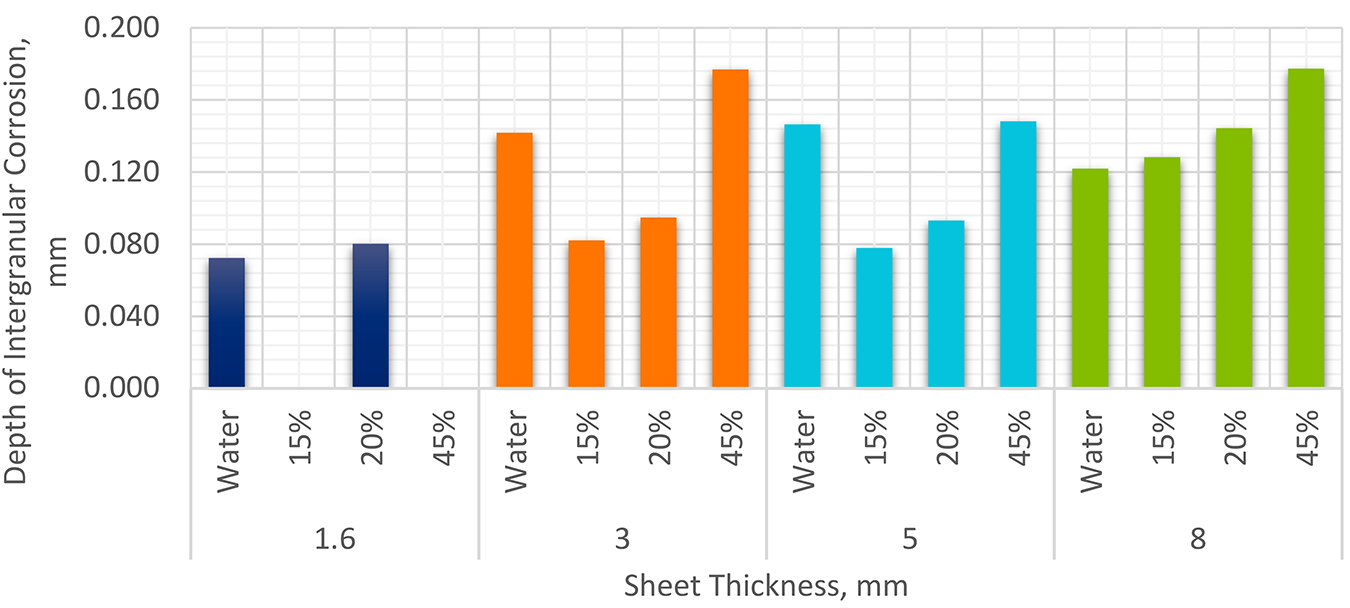
Conclusions
A series of panels of 2024 was heat-treated to the -T4 condition after quenching in water and different concentrations of an AMS 3025 Type I quenchant. In each case, with the exception of the 8 mm thick plate, the depth of intergranular corrosion measured was equal to or less for the polymer quenched panels than the water quenched panels. At lower concentrations, for the 8 mm thick plate, the Type I quenchant exhibited similar intergranular corrosion as the water quenched panels.
The use of polymer quenchants is very useful to reducing distortion and residual stress in quenched aluminum components while meeting mechanical property requirements and minimizing susceptibility to intergranular corrosion.
References
- SAE, “AMS 3025E Polyalkalene Glycol Heat Treat Quenchant,” SAE, Warren, PA, 2018.
- SAE International, “Heat Treatment of Wrought Aluminum Alloy Parts,” SAE International, Warrendale, 2015.
- X. Xiao, Z. Zhou, C. Liu and L. Cao, “Microstructure and Its Effect on the Intergranular Corrosion Properties of 2024-T3 Aluminum,” Crystals, vol. 12, p. 395, 2022.
- American Society for Testing of Materials, “ASTM G110-92(2015) Standard Practice for Evaluating Intergranular Corrosion Resistance of Heat Treatable Aluminum Alloys by Immersion in Sodium Chloride + Hydrogen Peroxide Solution,” ASTM, Conshohocken, PA, 2015.
- G. L. Kehl, The Principles of Metallographic Laboratory Practice, New York: McGraw-Hill, 1949.