Increasing demands and the need for higher performance mean the steel used in today’s applications has to be more reliable than ever before. New technologies like the ability to produce both high strength and high toughness in steel grades can make a meaningful difference in performance. To achieve these characteristics, consistent and effective thermal treatment is critical. That performance-based microstructure is achieved with a solid quenching practice.
The big picture: Factors that promote heat treating results
When designing a heat treatment, consider three factors:
- Material composition: Analyze the material’s ability to form martensite, a favorable microstructure in steel that’s used to optimize mechanical properties.
- Quenching medium: This should be coupled with the material composition to effectively and rapidly remove temperature from the material.
- Product cross section and shape: As the size of the product changes — increases or decreases — so does the temperature gradient over the cross section of that product.
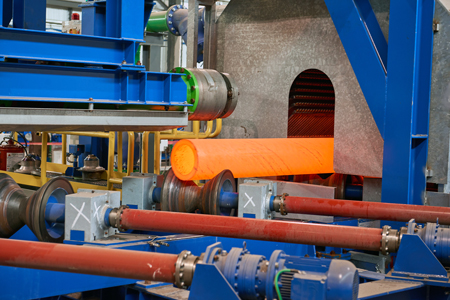
Why quench?
Simply, quenching steel makes it stronger. Quenching — the process of spraying water or other quenching fluid on heated steel or immersing the steel in a fluid — forms martensite, a hard, steel crystalline structure that results from the rapid cooling of the austenite form of iron. The martensitic reaction begins when austenite reaches the martensite start temperature and the austenite becomes mechanically unstable. As the steel is quenched, more of the austenite transforms to carbon-saturated martensite until the lower transformation temperature is reached. The shear deformations that result from the process produce a large number of dislocations, which ultimately strengthen the steel.
As quenched martensite is hard and strong, but not necessarily tough or ductile, tempering following the quenching process for any given chemistry provides the best opportunity for both higher strength and higher toughness in steels.
Components of quality quenching
Essentially, quenching provides the very foundation for high-performance steel; without it, characteristics like higher strength and toughness cannot be achieved. Several factors in the quenching process contribute to the steel’s reliability in demanding applications:
- Uniform heating: Maintaining a tightly controlled furnace temperature produces uniform mechanical properties and greater consistency from piece-to-piece, or even within a given piece.
- Fast transfer: To ensure martensite forms, it’s important to move the piece quickly from the furnace to the quench process to avoid premature, accidental formation of non-martensitic structures such as ferrite or bainite. The term slack quench is used when the product is delayed in transferring from the furnace to the quench, resulting in cooler product temperature prior to quench. Slack quenching is prohibitive to achieving high-performance mechanical properties.
- Adequate soaking: Having the ability to soak the entire length and cross section of the piece at the aim austenitizing temperature provides increased quality.
- Maintaining quench efficiency: Having a consistently heated product going into the quench is critical for effectiveness. This includes piece-to-piece and along-the-piece temperature control. Uniform temperature from front to back ensures better efficiency. Additionally, using the correct media (water, oil, polymer, etc.) is critical to ensure the quench practice extracts heat at the desired rates, as well as minimizes thermal stresses created during microstructure transformations. With a quench rate that’s too slow, the material may not achieve the desired microstructure to meet properties. In contrast, a rate that’s too aggressive can lead to undesirable thermal stresses, or worse, cracking of the components at stress risers.
- Uniform quench coverage: Uniform spray, like the unique, shell-shaped quench system offered with TimkenSteel’s advanced quench-and-temper facility, means the quenching fluid penetrates at the same depth for all product sizes. This removes heat and avoids the formation of a steam vapor barrier, which would prevent heat transfer from the piece. Uniform coverage also offers constant agitation, pressure, and flow throughout the process and can help maintain straightness of many products throughout the process.
- Uniform quench exit temperature/temper entrance temperature: Modeling is especially effective in identifying the right controls to achieve these temperatures. Large section sizes may quench to desired surface temperatures; however, the core temperature of the product may be significantly higher. This is typically seen in “rebound” where the product surface temperature will reheat after quench as the core temperature conducts back to the surface. Achieving uniform temperature post-quench ensures the desired microstructure through the full cross section of the component. Proper modeling can ensure the component is heat treating as desired.
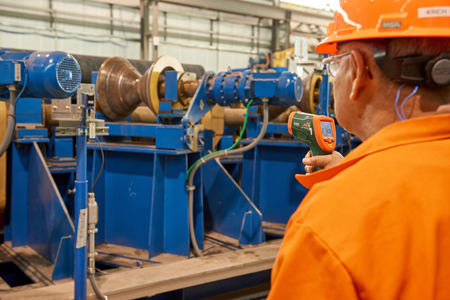
Process controls monitor effectiveness
To ensure heat treatment consistency, it’s important to have process controls in place that monitor what’s happening throughout each step. Some of these may include:
- Furnace modeling to guarantee piece-to-piece consistency.
- Thermocouples to monitor temperature uniformity within the zones.
- Infrared pyrometers to monitor the surface temperature of the product as it begins or completes critical stages of heating and cooling.
- Heat exchangers and/or cooling towers to maintain quench medium temperature control.
- Flow meters and pressure gauges to monitor quench uniformity and severity.
Proper controls promote the highest product quality by creating a reliable and repeatable process. These controls save time and money by allowing early notification of needed adjustments, as well as real-time adjustments at each step that eliminate the need for re-work.
Advanced modeling offers foresight
Consider the analogy of buying a car. When purchasing a vehicle, chances are you do a lot of research beforehand to ensure you’re getting one that best suits your needs. Advanced modeling in heating and quenching offers similar advantages in that it allows you to understand the overall process more thoroughly and to identify the best approach to apply heat treatment and how to correctly implement various process parameters to achieve the desired result.
Further, advanced modeling tools also may decrease the resources spent in quenching. Material models are extremely powerful in optimizing process design factors.
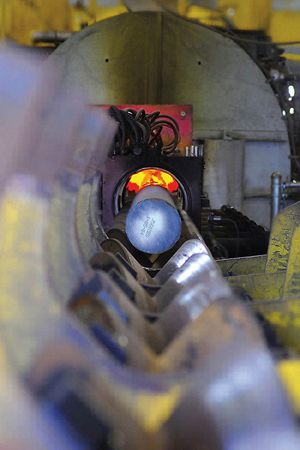
Hardenability reduces transformation
Steel hardenability refers to its ability to produce hard martensite at depths below the quenched surface. During quenching, the surface cools rapidly as the quenching aggressively removes heat. Further into the steel, cooling is limited by the steel’s ability to conduct the heat. In larger cross sections of steel, this slower cooling rate could allow the austenite to transform into a structure other than martensite.
Hardenability is related to the content of carbon and other alloy elements in steel, as well as the grain size of the austenite. The quenching fluid influences the cooling rate due to varying thermal conductivities. Hardenability is measured with a Jominy test: a round metal bar of standard size is fully transformed to austenite through heat treatment and is then quenched on one end with room-temperature water. The cooling rate is highest at the end being quenched and decreases as distance from the end increases. After the cooling is completed, a flat surface is ground on the test piece, and the hardenability is determined by measuring the hardness along the bar. The farther away from the quenched end the hardness extends, the higher the hardenability.
The Jominy specimen is typically tested using Rockwell C hardness at specified distances from the quenched end. Each of these test distances correlates with a cooling rate, which can be superimposed onto the cross section of a component. Jominy tests for a given lot of steel can therefore be used to predict microstructure and as-quenched properties through the cross section.
The goal of any quenching process is to form martensite throughout the entire cross section, or at least to form martensite in all parts of the cross section where high strength and high toughness are required.
Figures 1 and 2 are called continuous cooling transformation (CCT) curves. These time (logarithmic scale) vs. temperature curves help describe how hardenable a given steel is, and what structures can form in steel as a function of cooling rate. The light blue curve annotated with arrows in Figure 1 is the approximate time vs. temperature during cooling at the center of a 6-inch diameter, water-quenched bar. It takes a little more than 300 seconds — or 5 minutes — to get the center to cool by about 100 degrees Celsius. At about 6×10 seconds (60 s), the center reaches 640 degrees Celsius and ferrite begins to form. As cooling continues, more ferrite forms until the center of the bar reaches about 360 degrees Celsius, at which point the remaining austenite transforms to martensite.
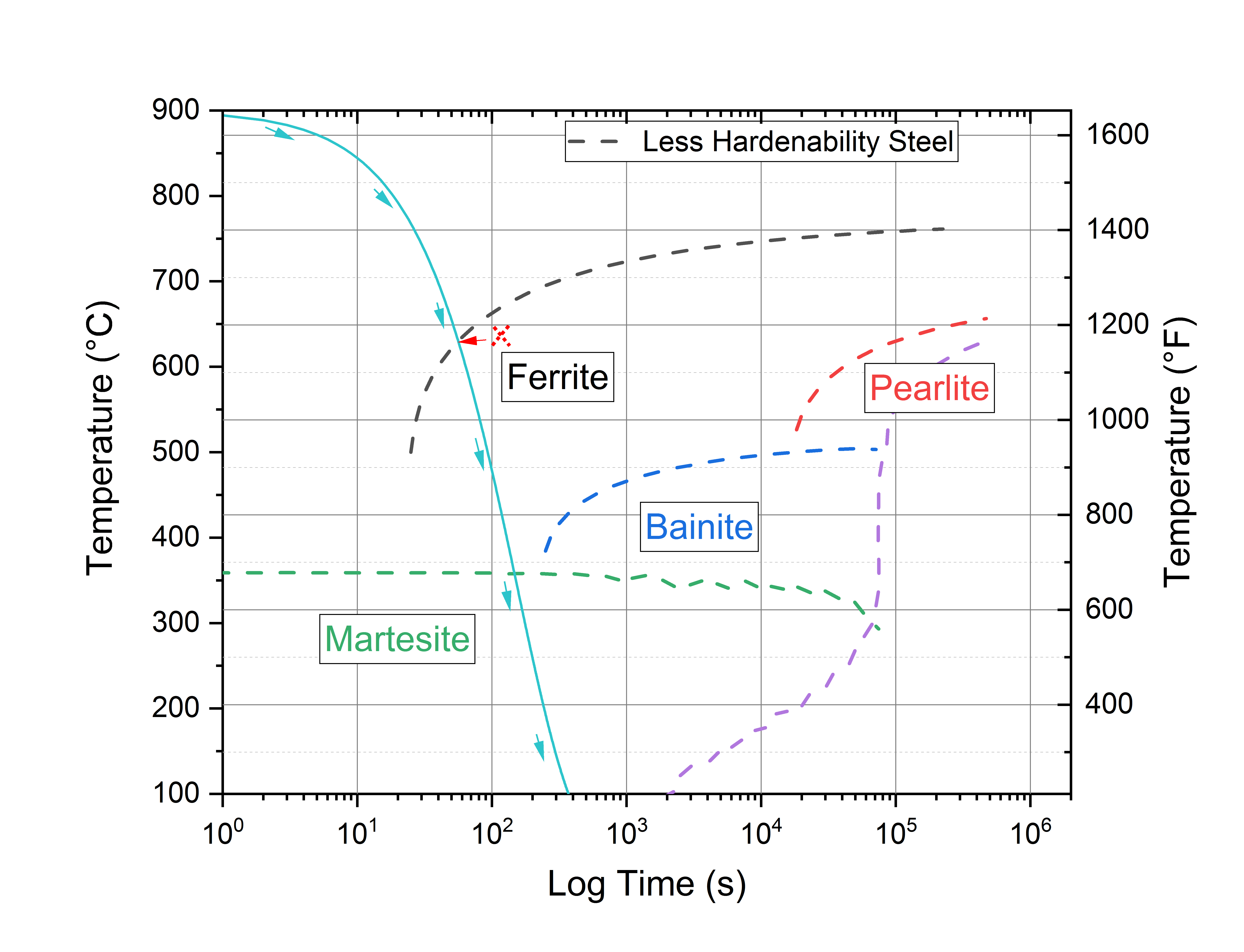
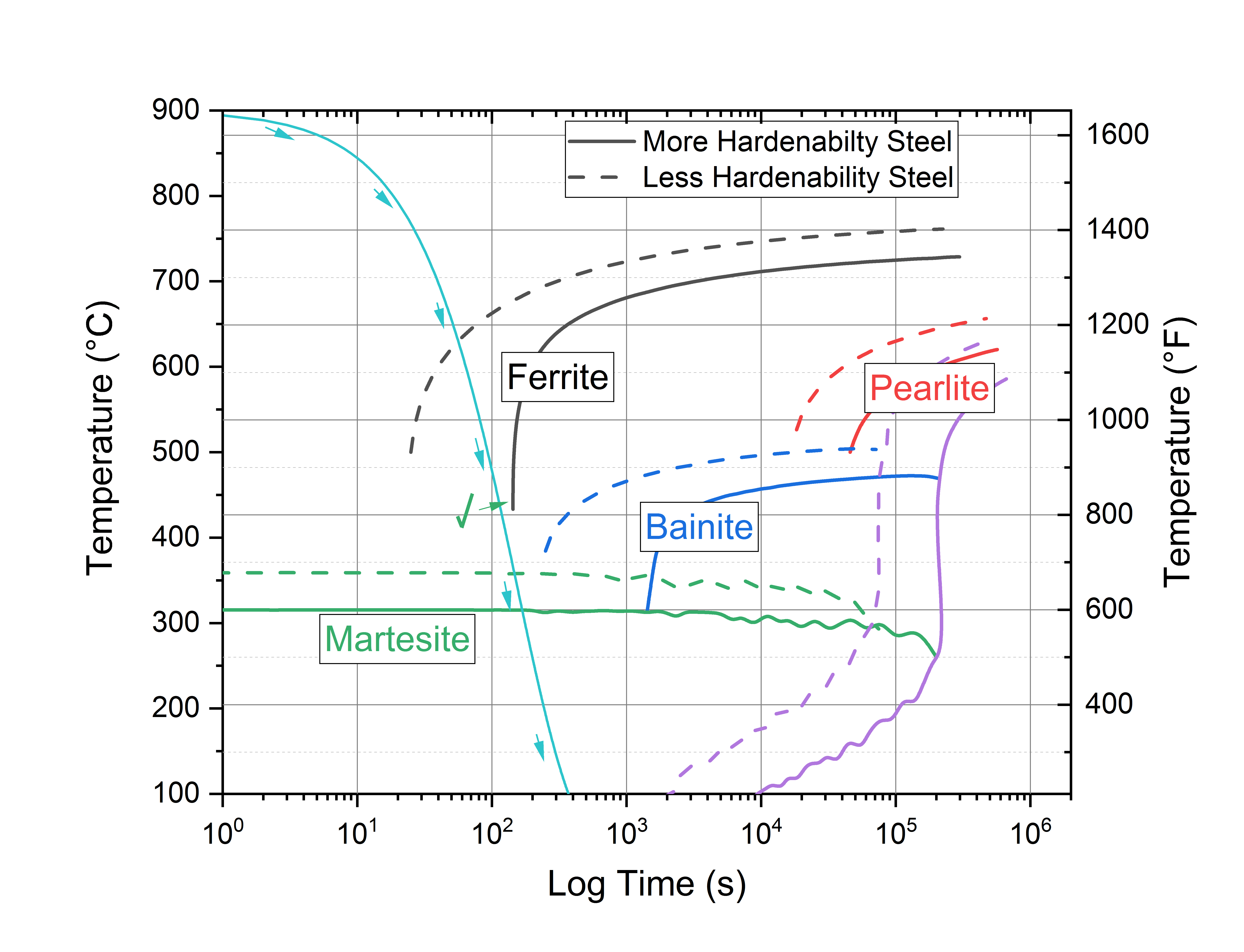
Now, consider Figure 2. Here, a second set of CCT curves is included for a steel that has higher hardenability. This higher hardenability is achieved with the addition of alloying elements that delay the ferrite, pearlite, and bainite transformations, causing the CCT curve to be pushed to the right to longer times. Here, the same cooling path now misses the “nose” of the ferrite transformation curve and only martensite is formed at the bar center.
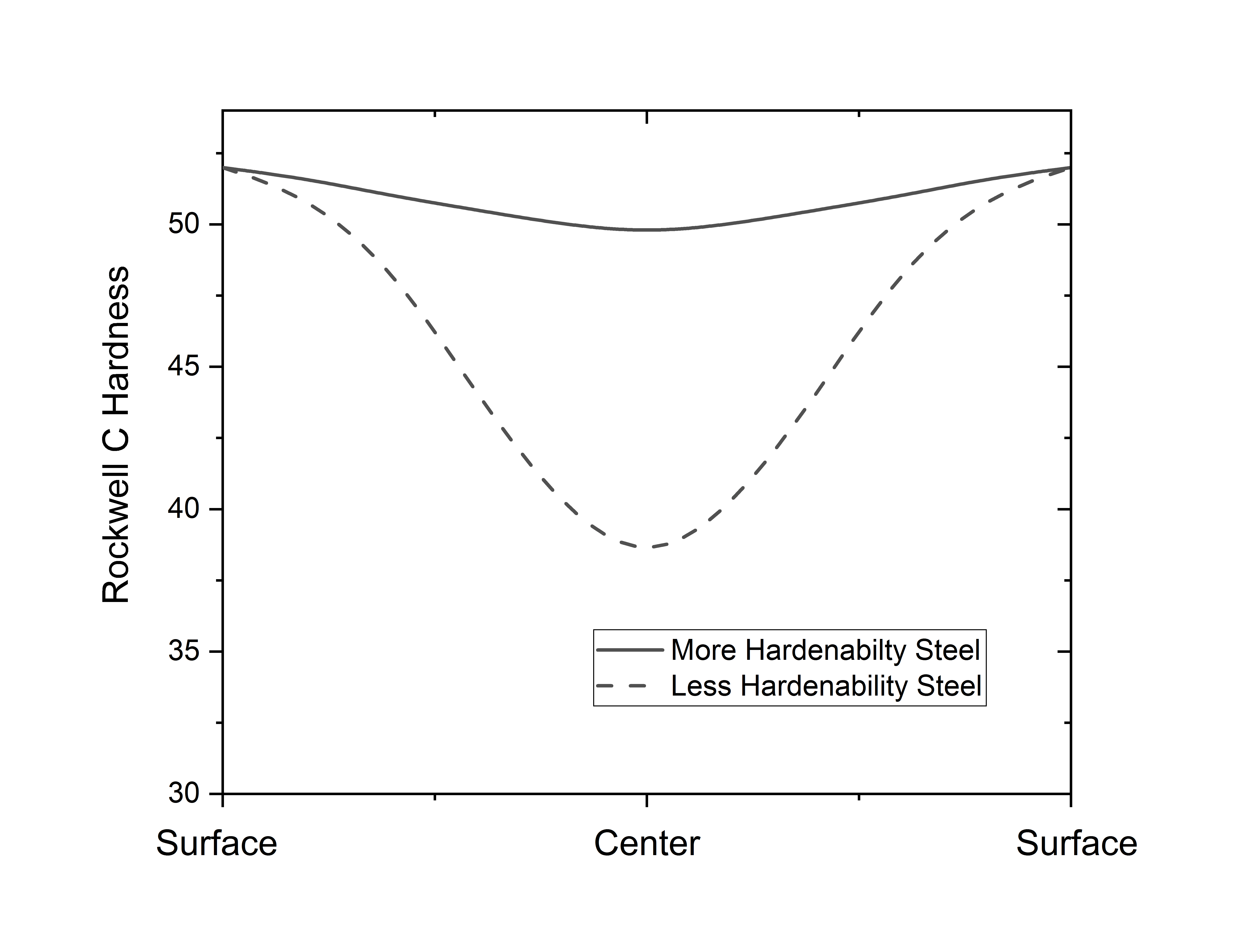
The difference in hardness associated with these illustrations is shown in Figure 3. A higher-hardenability steel allows you to achieve high hardness throughout the cross section.
Application to batch processing
The above discussion focuses primarily on continuous or in-line quenching. However, many of the same principles apply to batch and component processing. Variables such as fast transfer, monitoring quench medium temperature, constant agitation, and limited load for uniformity are all important in guaranteeing a high-quality end product. Both batch and component processing present additional, unique challenges.
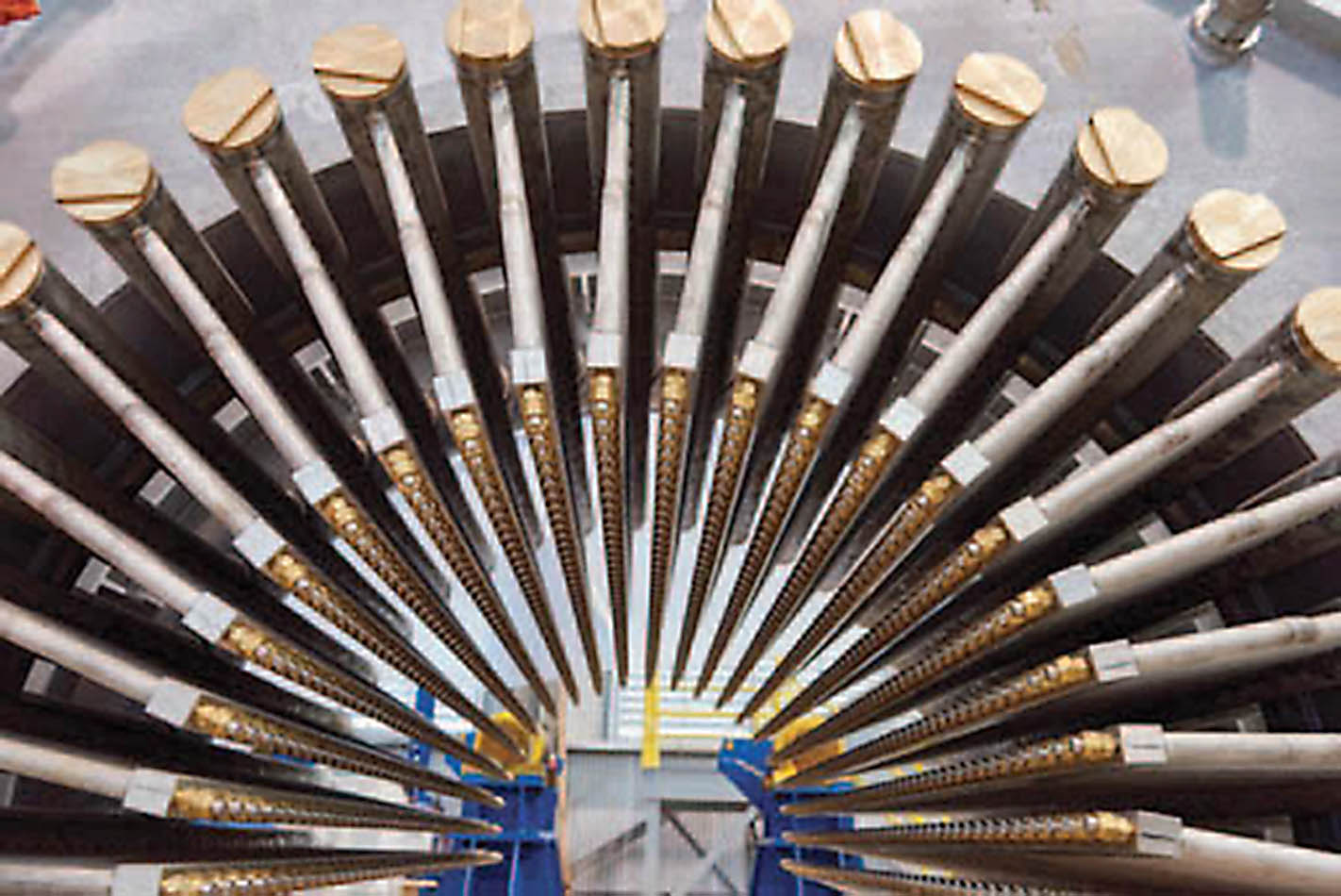
With good quenching and tempering practices, the real benefit to any steel customer is an end product that’s more reliable, with the ability to achieve higher levels of performance. Following a solid heat treatment path means steel is stronger, tougher, and ultimately more capable, which may translate to higher loads or better endurance at existing loads, increased fatigue strength, wear resistance, and resistance to bending overload damage.
The classical trade-off in all materials is that increasing strength nearly always results in reduced toughness. But, with careful design and calculated processing — including thorough quenching and tempering — today’s steels can achieve significant strength improvement with excellent toughness properties. For customers, that means enhanced solutions for demanding applications.
Learn more at www.timkensteel.com/what-we-make/specialized-services/thermal-treatment.