As the demand for additive manufacturing (AM) products has steadily increased, so have the many enabling technologies including laser powder bed fusion, laser metal deposition (LMD), wire arc additive manufacturing, thermal spraying, and electron beam melting (EBM). All of these rely — directly or indirectly — on industrial gases such as nitrogen, argon, and helium.
Although AM processes still constitute only a fraction of powder-metal (PM) parts applications, the AM sector is growing and is of special interest due to a number of unique advantages: freedom of design and alloying, short lead times, no need for additional tooling for different parts, and virtually 100 percent use of materials. At the same time, AM production processes are generally limited to certain alloys, parts of moderate size and weight, and to short series runs because processes are slow.
Medical and aerospace parts that can live within or leverage these limitations are currently the primary applications for AM processing. In the aerospace industry, these are mainly non-critical, low-stressed “static parts” made from traditional construction materials such as Ti6Al4V and Inconel 718, a high-strength, corrosion-resistant nickel chromium alloy. However, engine fuel nozzles, for example, are in serial production at both GE and Airbus AM manufacturing plants. In the medical and dental area, AM products include standard implants such as hip joints, external prostheses, hearing-aid shells, and dental crowns and bridges.
The automotive industry is also ramping up to a more prominent role. Advanced and complex parts produced in short series such as for racing cars, as well as certain spare parts, tools, and prototypes are a few examples. Up to now, automotive original equipment manufacturers (OEMs) and suppliers primarily used AM for rapid prototyping. Yet the pace of AM development coupled with quality improvements and machine redundancy are now making AM a candidate for direct manufacturing of some parts beyond a limited scale.
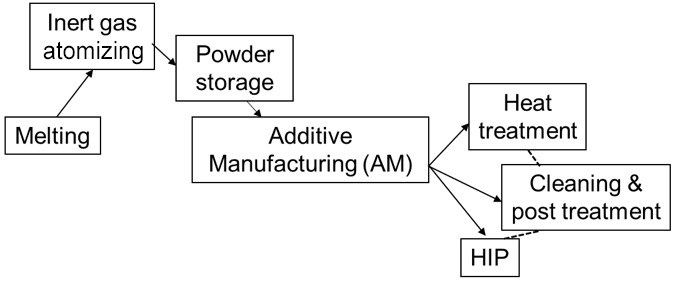
Industrial gases already play a crucial role in several PM process steps such as in inert-gas atomization of metal melts, as a pressure medium in hot isostatic pressing (HIP), and as a controlled atmosphere to avoid oxidation and to maintain carbon content in sintering steels, etc. This paper focuses on steps that use industrial gases before, during, and after AM as summarized in Figure 1.
Melting and Atomization
Induction vacuum melting is the preferred production process for powders to be used in AM. The melt is atomized with a high-speed jet of inert gas that creates fine metal droplets that cool as they fall into the atomizing chamber. A finer particle size is required for AM powders than for HIP or cold pressing and sintering. One reason is that finer particles improve powder flowability, an important parameter for AM processing. Sufficient flowability allows the roller to build up thin layers (see next section) in the powder bed chamber. If flowability is too low, it decreases the resolution of the fabrication process due to insufficient recoating. On the other hand, very high flowability does not provide sufficient stability for the powder-bed process. The proper gas pressure and nozzle design are required for generating the right size and form of atomized melt droplets to meet these demands. (Figure 2)

The inert gas disintegrates the melt into spherical powder particles and also protects the particles from reacting with air and oxidizing. Accordingly, the inert gas must be of high purity. Nitrogen is used with most metals or alloys. Some require argon and, in a few cases, helium. Argon is used when the powder material tends to pick up nitrogen, such as with titanium and certain high-chromium alloys, and including stainless steels. These materials can be inadvertently nitrided, making them brittle or, in the worst case, useless. Helium is used as an atomizing gas where its outstanding thermal properties can play an important role.
Strength and ductility of parts processed from powder will increase with decreased oxygen content in the powder. Therefore, a purity requirement should be valid for the gas supplied to the atomizing chamber, typically below 10 ppm. But even more importantly, there should be a purity requirement within the process chamber.
Powder Storage and Quality Control
Screening and classification of the powder follows atomization, and then packaging or storage. Powder is typically stored on an open shelf or in a closed cabinet somewhere near the AM equipment until it is needed. Oxygen in the ambient air and humidity in storage can negatively affect the chemical and physical properties of the powder. This is particularly true of sensitive powders such as aluminum and titanium alloys. Because the powder fractions used for powder-bed AM are much finer than traditional PM, HIP, or sinter powders, AM powders are especially vulnerable.
The ADDvance® powder cabinet addresses this storage issue with an atmosphere monitoring and control system that actively combats air infiltration and humidity. It works by continuously measuring the humidity level inside the cabinet and uses a stream of purge gas to maintain a non-critical moisture value. Whenever the cabinet doors are opened and humidity rises, ADDvance triggers a high-volume purge gas flow as soon as the doors close again to rapidly remove moisture in the air (Figure 3). It then applies a lower stream of gas to ensure a consistently low level of humidity. The control sequence is repeated whenever the doors are opened and closed to add or remove powder.
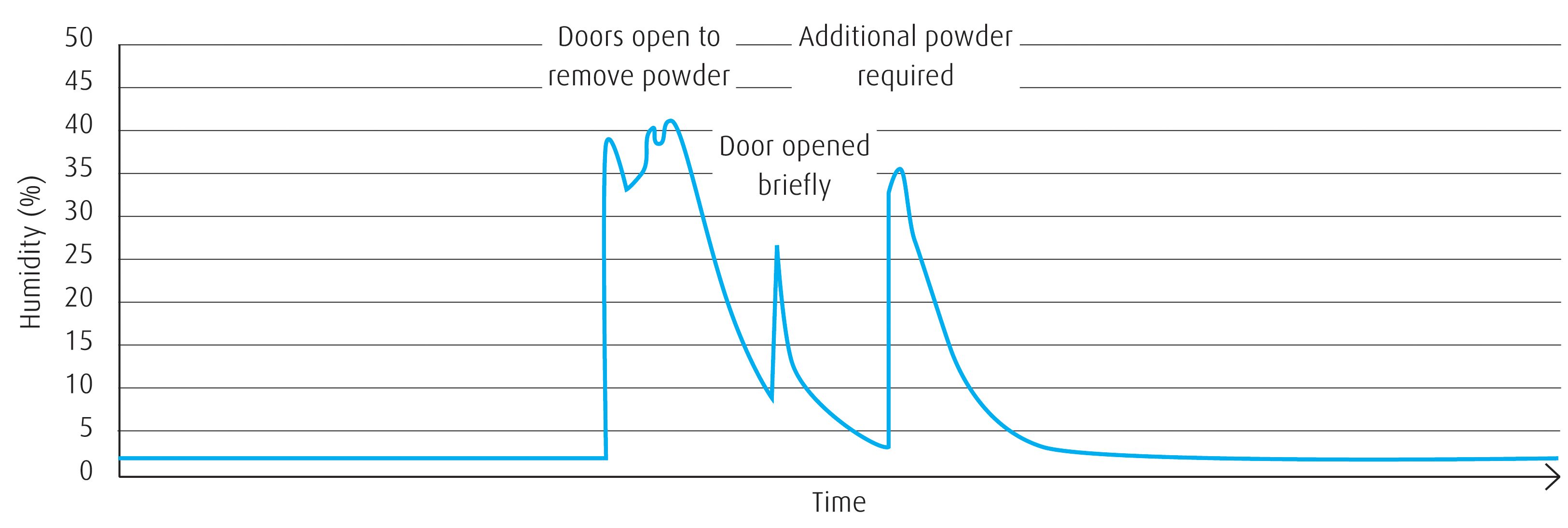
ADDvance uses a directional gas stream and a carefully selected flow rate to ensure the desired moisture value. The system is economical since it does not waste purge gas when the door is open. It only triggers the purge stream once the doors are closed again.
Quality AM Processes
There are different several basic methods of metal additive manufacturing, but one of the most common uses a powder bed that is selectively heated with a laser or an electron beam. These include laser powder bed fusion and EBM.
The heat from the laser or the electron beam will sinter or melt the powder particles together, successively as shown in the left part of Figure 4, such as laser powder bed fusion. At the start of the production process the building platform is covered with a powder layer. A laser beam then is moved to sinter or melt the powder onto the object form. When that layer is fused (sintered or melted), then the building platform is lowered, and a new layer of powder is added and fused. This sequence is repeated until the object is completed.

The process chamber is filled with inert gas, nitrogen, or argon, to avoid detrimental oxidation of the heated powder. As with atomization, it is important to define a purity for the gas supplied, typically below 10 ppm, but even more important to define a purity requirement within the process chamber. With the ADDvance® O2 system, it is possible to define and maintain the oxygen (O2) concentration inside the chamber to the level required by material and application (Figure 4). The system continuously analyzes O2 levels in the process chamber to concentrations as low as 10 ppm. It automatically initiates a purging process to keep the desired atmosphere concentration level in case the atmosphere is disturbed. The system also monitors dew point and indicates trace levels of hydrogen.
As an alternative to using an inert gas atmosphere, it is possible to tailor the gas composition in the process chamber to actively influence the material to attain certain properties. Electron beam fusion, for example, uses helium in the process chamber because of its favorable thermal characteristics.
An alternative to building up parts in a powder bed is to supply material as a powder or from a wire that is melted and deposited onto the object (Figure 5). Laser Metal Deposition (LMD) and Electron Beam deposition are two of these methods. The powder is contained in a carrier gas and directed to the substrate through a nozzle. Alternatively, a wire can be fed from the side. The powder or wire is melted to form a deposit that is bonded to the substrate and grown layer by layer.

An additional gas jet, concentric with the laser beam, provides additional shielding or process gas protection. This method is suitable for larger components where a higher deposition rate is required. LMD is used for a range of applications including cladding and repair of high-value parts such as aerospace engine components and military equipment.
HIP, Heat Treatment, and Cleaning
There are high demands for reproducible parts quality in medical and aerospace applications. Post-HIP treatment, therefore, is used almost without exception for such AM products to achieve full density and freedom from defects. HIP takes advantage of the possibilities of simultaneous high pressure and high temperature in the production of parts from metallurgical powders. The equipment (Figure 6) comprises a furnace chamber enclosed in a pressure vessel adapted to withstand extremely high pressure and which can also be fully evacuated to vacuum levels. The processes are applied at different pressures and temperatures depending on the materials involved. HIP equipment is available with operational pressures up to 200 MPa (29,000 psi) and temperatures to 2,000 °C (3,600 °F). Gas is indispensable for HIP, and argon or nitrogen are used as the pressure medium.
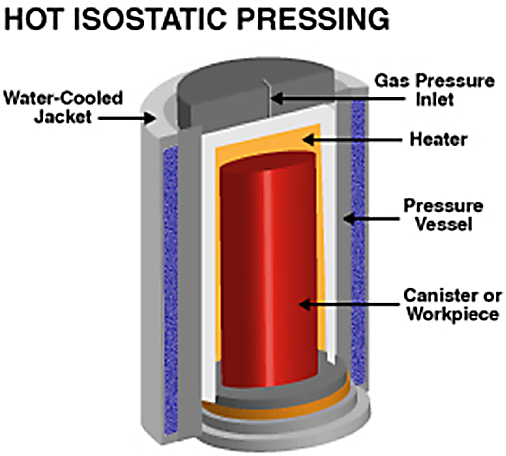
Due to the steep thermal gradients created during AM, residual stresses remain in the parts after processing, so a stress relieving treatment is usually required. In addition, a columnar grain structure normally results from the repetitive layering of powders melted or sintered onto the surface. A heat treatment can restore the microstructure. Other post heat treatments may also be required to give adequate strength and toughness of AM produce parts. Hardening, carburizing, or nitriding are examples of the processes that may be required. Nitrogen, argon, hydrogen, specialty gases, and methanol are necessary supplies in these processes.
Linde offers a number of proprietary atmosphere control systems for annealing, hardening, carburizing, carbonitriding, nitriding, nitrocarburizing, and sintering, under the tradenames HYDROFLEX®, CARBOFLEX®, CARBOJET®, NITROFLEX®, and SINTERFLEX® atmosphere control systems and solutions.
AM parts exhibit a certain surface imperfection with excess powder adhered to the surface after processing. Linde has developed the ADDvance® Cryoclean, a patented process for cleaning with the help of CO₂. The device creates dry ice particles by expanding liquid CO₂ directly. Using compressed air, the particles are accelerated in a de Laval nozzle up to sonic speed and shot onto the surface to be cleaned. The cleaning effect of this procedure relies on flash cooling, kinetic energy, embrittlement, and gas impact. An abrasive agent can be admixed with the dry ice particles to remove stubborn powder residue. It enables the operator to adapt the CO₂ snow/abrasive material ratio from gentle to abrasive. With this process, much less abrasive material is needed to remove adhering powder residue compared to conventional sand blasting.
ADDvance Cryoclean is a specially designed cleaning unit for AM metal components. The encapsulated equipment design provides maximum health protection and safety for the operator. Alternative cleaning methods such as grinding or chemical cleaning are harmful to the environment and less productive.

Safety
Metal powders are not just sensitive to atmospheric influences, but they can pose hazards to human health and safety. Careful storage of powders in a blanket of inert gas such as nitrogen is a basic safety measure that also reduces risks for dust explosion or fire. Products and services provided by The Linde Group to customers around the world must meet high quality and safety standards. This applies not only to the “end” product or service, but to the company’s whole value chain from gas production and supply to the commissioning of gas application technology — and safety training is part of every installation.
In the final analysis, successful PM production, including advanced AM processes, rely on the proper use and safe handling of industrial gases. So, the future of additive manufacturing will depend, in a very real way, on the quality application of gas technology to key facets of production — from supply and storage of materials, to atmosphere monitoring and control systems, to heat treatment and finishing of final parts.
For more information, contact Linde LLC (www.lindeus.com), 1-800-755-9277.