Any article on pyrometry, as it applies to AMS2750 and thermal processing, usually gets a lot of attention. Typically filled with interpretation, clarification, and general discussion, it is understandable that pyrometry can be difficult to interpret at times, especially for someone new to the topic. Using my own experience in the industry, I have written plenty of articles that address specific topics in pyrometry attempting to convey my interpretation as well as suggestions on how to accomplish testing and conformance based on my own experience in the industry. This typically yields reader feedback and, most importantly, questions regarding more specific topics as well as questions about my interpretation. In the end, this allows discussions in which both sides learn something, and, hopefully, something positive is produced.
On rare occasions, I get asked, “Does it really have any effect on my specific product?”; as I do not have design authority over the specific product in question, it is not my place to answer with any authority, only my professional opinion. Conversations face-to-face regarding pyrometry (or nonconformances in pyrometry) tend to produce this question as well (and will usually give the same answer). The answer given is not to dodge the question in any way but is the only fair approach in those situations. While we may not be able to answer the “Does it really have any effect on my specific product?” question, what we can do is look at how pyrometry may alter the structure of parts processed in a furnace overall. I will also include some of the upcoming revisions in AMS2750F and explore how those specific changes may alter the structure of metallic materials processed.
Thermocouples
Microstructure changes due to heat treating is dependent on, among other things, heat transfer, whether direct or indirect and its subsequent cooling. Believe it or not, the subject of heat transfer can get quite involved when you start diving into the technical side. This will include plenty of formulas (including the 1st law of thermodynamics), which will explain the nonstationary temperature fields developed when heating and cooling over time. For now, we will not dive deep into this. The point being that heat transfer is an important part of any heat-treat process. Some industry specifications require that parts be held at temperature for, say, “30 minutes per 0.5” of material thickness.” This is a consequence of heat transfer. If a material is not held at temperature long enough, the product will not reach the desired temperature throughout and, therefore, will not have homogeneous properties throughout. Product temperature is measured using thermocouples, which is covered extensively in AMS2750.
Load thermocouple usage and replacement is described in AMS2750 in two categories: expendable and nonexpendable. The usage categories are appropriate for both types in that, if a thermocouple has only fiber or plastic shielding, it is not as well protected as a metal sheathed thermocouple where the hot junction is not exposed. The limitations for thermocouple use are based on the fact that thermocouples degrade over time when cycled up and down in temperature. Atmosphere also affects the degradation of thermocouples. If the thermocouple degrades enough, it loses the ability to measure part temperature accurately. If a thermocouple is not reading the correct temperature, a process time may be started long before the part temperature actually reaches the desired point. This would potentially cause the properties of the material to be nonhomogeneous throughout. It would be difficult to detect this issue with hardness testing alone. A more destructive form of hardness testing would be needed. (See Figure 1)

Whether the degradation of thermocouples happens at a rate that merits the usage restrictions in AMS2750E and the upcoming more stringent requirements in AMS2750F is arguable based on many process variables as well as a lack of peer reviewed and repeated tests.
Temperature Uniformity Survey
This is, in my opinion, the portion of AMS2750 that will have the most dramatic effect on product processed in furnaces. Let’s begin by taking a look at how poor uniformity in a furnace can affect the properties of aluminum during solution heat treating.
By definition, heat-treatable aluminum alloys are those that can be strengthened by a suitable thermal process for that particular material — for example, alloys that contain magnesium (Mg), as the addition of Mg provides solid solution strengthening without decreasing ductility. In general, solution heat treating takes advantage of the precipitation hardening reaction. Its objective is to take into solid solution the maximum practical amount of the soluble hardening elements in the alloy. This process also consists of soaking the alloy at a temperature sufficiently high and for a long enough time to achieve a nearly homogeneous solid solution. To obtain the maximum concentration of magnesium and silicon, the solution temperature must be as close as possible to the eutectic temperature — ideally 10-15°F below the eutectic temperature. Control of temperature is critical; if the melting point is exceeded, incipient melting (localized melting at the grain boundary) (Figure 2) may occur and mechanical properties may suffer. This condition is only detectable by metallographic examination and is irreversible. This is why furnaces used for aluminum solution heat treating are typically required to have a temperature uniformity of ±10°F or better.

Now we will look at vacuum brazing as an example. More specifically, a diffusion braze cycle that, after the standard braze cycle, is held at a temperature just below the solidus temperature that is intended to reduce the opportunity for alloy re-melt (and subsequent failure of the joint) once the product is put into service. Let’s assume I have five samples on a ceramic plate in a vacuum furnace that I intend to put through this cycle. Each corner has a sample, and one sample is placed in the center of the ceramic plate. The goal is to process the samples in a furnace that will produce the same microstructure in each piece. Let’s assume the cycle is as follows1:
1. Ramp to 1,750°F ±15°F and hold for 15 minutes.
2. Ramp from 1,750°F to 2,025°F ±10°F and hold at 2,025°F for 6 minutes.
3. Vacuum cool from 2,025°F to 1,800°F ±10°F and hold for 1 minute minimum.
4. Gas fan cool from 1,800°F to ambient.
No. 2 is the standard braze step and No. 3 is the diffusion braze step. If the required uniformity of ±10°F is not achieved within the furnace at the time of the braze cycle, neither braze step will produce a conforming microstructure in the samples. In the end, the microstructure of the nonconforming part will show a lighter diffusion zone (1), minimal solid solution loops (2), and a larger eutectic matrix (3) (see Figure 3).
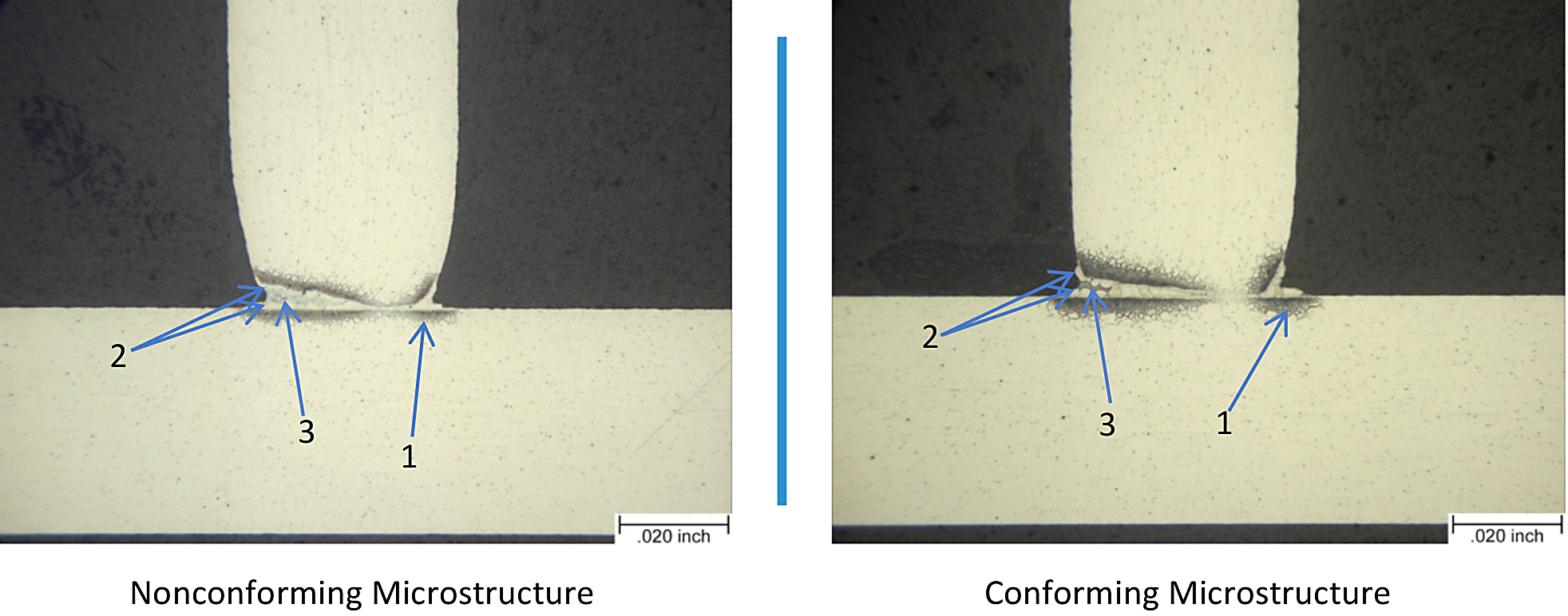
With these two examples, we can see the importance of temperature uniformity cannot be understated. It is apparent that, the more sensitive the metallurgical process is, the more stringent the uniformity requirements will be.
Instrument Calibration and System Accuracy Testing
We all want our records to show accurate temperature readings. Our customers and auditors want to see this as well. An instrument calibration ensures the furnace and/or test instrument meets the accuracy requirements stated in AMS2750E when compared with an instrument of greater accuracy. A system accuracy test ensures that the entire furnace system (instrument, lead wire, and sensor) used, when compared to a test system (instrument, lead wire, and sensor), meets the tolerance of AMS2750 (Figure 4).

It is important to recognize that instrument calibration and system accuracy testing is not the same test. I am sometimes asked to explain why the two tests are performed if they “seem to be the same; testing the accuracy of the readings.” This is not the case.
An instrument calibration is performed by transmitting a simulated mV reading into the instrument. If the alternate SAT is employed, those specific channels on the recording system must be calibrated at the point where the furnace thermocouple is connected. The simulated reading is coming from an instrument that has greater accuracy than the instrument being tested and should, theoretically, enable the user to identify that the accuracy of the furnace instrument is within the limitations of AMS2750.
A system accuracy test uses a test system more accurate than the furnace system. The key to this test is that a system is being tested, not just a thermocouple or an instrument. It is important to think of each as a system. In other words, an SAT is not being performed on the control, but the control system. This is apparent in the SAT section of AMS2750 as it states multiple times that the system includes the instrument, lead wire, and sensor (see Figure 4).
If the instrument being used (i.e. control and/or recording system) is not reading the temperature of the furnace/parts correctly or if the furnace control/recording system is not accurate, the product being processed may not achieve the metallurgical transformation intended. There is a correlation between the permitted SAT difference and the temperature uniformity. This is shown clearly in Tables 6 and 7 of AMS2750 (see Figure 5). The tighter the furnace class, the tighter the SAT difference. Or, put another way, the more sensitive the microstructure is during thermal processing, the tighter the uniformity and SAT difference allowance.

AMS2750F Proposed Changes
I won’t be going into every change proposed for AMS2750F. I will, however, take a look at some proposed changes and discuss how those items may affect the microstructure of product processed in a thermal cycle. I would like to use this opportunity to state that, in general, the proposed changes to AMS2750 are logical, and quite a bit of the Nadcap pyrometry reference guide is being incorporated into the specification, which I see as a positive move.
Thermocouples
It is proposed that expendable base metal (excluding Type N) SAT, TUS, and load thermocouples be limited to a single use above 500°F. This will be a major change for most processors and, inevitably, cause an increase in operating cost that will, no doubt, be flowed down to consumers in the end. As discussed earlier, the degradation of thermocouples over time must be controlled to ensure the readings obtained during processing are accurate to ensure the product has achieved the correct temperature for the required time. This change is based, in part, on a study that examined the drift in Type K thermocouples in an oxidizing atmosphere2. It could be argued that the new expendable base metal (excluding Type N) thermocouple usage limitations proposed for AMS2750F are not in line with the experimental data, as the experiment used an oxidizing atmosphere, and the changes will apply to all atmospheres (i.e. vacuum, inert atmosphere, etc.).
Instrumentation
Furnace instruments will potentially need to be calibrated within ±1 minute/hour. This proposed requirement is especially important for those that process very short cycles, such as high temperature nickel brazing. Some Ni braze cycles can be between 4-7 minutes, making the timing function critical to the metallurgical transformation process. All of that aside, it is just good practice to calibrate the timer being used to certify the process run time.
System Accuracy Testing
A proposed change to the SAT section is for furnaces with multiple qualified operating ranges to have a periodic SAT performed in each range at least annually. As stated, SAT measures the accuracy of the entire system being used to certify the product was a) at the correct temperature and b) for the correct time. The permitted SAT difference changes depending on the uniformity requirements (furnace class) for that particular range. That being the case, if a furnace is qualified, say, at ±25°F from 1,000°F to 1,800°F and ±5°F from 1,801°F to 1,950°F, the system would need to be more accurate at the higher range. This proposed change for AMS2750F will ensure processors account for each range and its relative permitted SAT difference. From a metallurgical perspective, this will ensure the required transformation in a more sensitive material occurs and conforming results are obtained.
Another potential change within the TUS section will require that, for vacuum furnaces using both vacuum and partial pressure during production, the furnace must be tested at least annually within the partial pressure range used during production. This is in addition to the periodic testing at vacuum. There is potential for uniformity variance once partial pressure is introduced. This will typically occur at the point where the gas enters the furnace. If a furnace is tested at vacuum and achieves, say, a +6°F/-3°F uniformity and the requirement is ±10°F, it will pass. If partial pressure is then introduced on select cycles, there is a chance the uniformity will vary. The uniformity may change drastically enough to where the furnace is unable to hold a ±10°F due to the inlet of the partial pressure gas used. As discussed earlier, nonconforming temperature uniformity can have a major effect on the microstructure of materials processed. While this requirement is logical, it could be argued that the frequency of this test should be treated like radiation surveys in that, if there is no mechanical, type, or flow changes to the delivery system, why perform the test annually?
Offsets
This topic, in my experience, has previously produced quite a bit of ambiguity — not anymore. The proposed changes make the practice of implementing and using offsets as clear as they could be made. Of course, it is only my opinion, but the changes made to the offsets section is, from my view, the most well thought out and comprehensive changes made. As always, there are always varying opinions, especially on this topic. I will not be going into the details of the (what I see as) positive changes to offsets as it would take up the better part of a publication. Offsets, if not controlled, can have a negative effect of the quality of products’ microstructure. A combined offset amount within a control can be rather high, depending on the class of furnace, and, if not controlled and implemented correctly, will in turn indicate an inaccurate temperature. This scenario could cause the thermal cycle to start the soak time too early and not allow the microstructure to achieve the desired results.
Summary
The “Does it really have any effect on my specific product?” question should be revisited at this point. While we can explain, in general, how aspects of pyrometry affect the microstructure of product, we cannot use that as justification to deviate from requirements. One may not feel that 0.5°F above the tolerance during an instrument calibration has any detrimental effect on product; it is, nonetheless, nonconforming and must be addressed. While considering the metallurgical effects of the different aspects of pyrometry is a useful tool in understanding pyrometry from a cause and effect point of view, it is incumbent on the processor to ensure conformance to all aspects of pyrometry and Nadcap. This can only come from continuous training and experience.
Footnotes
1 User assumes all risk when attempting to repeat this cycle.
2 E.S. Webster — Drift in Type K Bare-Wire Thermocouples from Different Manufacturers. 2017.