The greatest hurdle in the production of powder metal components is lubricant removal. Over the years, equipment and processes have been developed to aid in the removal of the lubricant during the sintering process. Unfortunately, these technologies were not successful in removing all the lubricant.
Recent developments in the sintering process have resulted in a paradigm shift of the way lubricant removal is addressed. This new technology is called the Vulcan process. A process and accompanying equipment, the Vulcan has demonstrated the ability to remove all the lubricant from the compact, the result of which is the increase of physical properties of the sintered part. Transverse rupture strengths can be increased up to 18.0%. Hardness can be increased up to 6.3%. Density can be increased by up to 1.7%. The performance of the Vulcan is also demonstrating a significant opportunity to reduce the cost of production without compromising quality.
Introduction
Lubricant is needed for the compact to be ejected from the compaction press. The industry has worked to reduce the amount of lubricant that is used, but some lubricant is always needed. The problem occurs during sintering. The particles need to be in contact with one another or physical properties of the component will be detrimentally affected. The once-needed lubricant and oxide from the manufacturing of the powder act as barriers between the particles. Therefore, sintering steps need to remove these barriers to improve the physical properties of the component.
The steps begin with the heating of the compact. This allows the lubricant to melt and be wicked from the part through capillary action. If the lubricant is not removed from the compact, a carbon residue will form and affect the final properties of the product. Next, the atmosphere needs to be manipulated to react with the oxide. Hydrogen (H) is injected to react with the oxide (O-2) forming water H2O, removing the barrier from the particles. The clean particles of powder are in contact with one another, allowing for a better sinter, resulting in increases in physical properties of the component.
The workhorse of lubricants used in the industry is Acrawax CTM. This is an N,N’ ethylene bis-stearamide-based synthetic wax (EBS) with a melting point between 284°F to 293°F (140°C to 145°C) [4]. In work done by Powell, Stringer, and Feldbauer, it was shown the optimal lubricant removal temperature for EBS is between 350°F to 1,000°F (177°C to 537°C) [5]. This temperature range is relatively low when compared to sintering temperatures. Temperature control at this low temperature requires convective heating to maintain the low temperature.
Also, in work by Powell, et. al., it was shown if lubricant is exposed to temperatures above 537°C (1,000°F), soot is formed [5]. This sooting causes carbon deposits internally and externally of the part. This carbon contamination in the part causes variations in properties and contamination within the furnace itself.
Until recently, it was thought lubricant exited the compact early in the process, and a “rule of thumb” was 20 minutes for an iron-based compact. In work by Powell, et. al., it was demonstrated weight loss stopped in approximately 22 minutes for parts of 6.2 g/cm3 density [5]. However, compaction technologies have made significant improvements since then. The typical density of an iron-based product today is above 6.8 g/cm3, and it is now common for producers to have densities as high as 7.5 g/cm3 for some products. Also, until recently, it was thought that, with increased density, lubricant removal temperature needed to increase, the opposite of what was described earlier.
In the paper by Powell, et. al., the time to remove EBS as a function of green density was measured, and a mathematical model was developed [5]. The model shows that, with increases in green density, lubricant removal times increase exponentially. Also, as part geometry grows, the pathway for the lubricant to exit the part increases. This requires the component to be in the optimal lubricant-removal-temperature range longer. So, the results of bigger/higher density parts containing EBS are significantly longer lubricant removal times.
In work done by Levanduski and Feldbauer [2] and verified by work done by Powell, et. al. [5], it was shown the hydrocarbon EBS unravels as the temperature increases in the optimal lubricant removal temperature range. The work shows that, as temperature increases, EBS unravels until the simplest hydrocarbon, methane, is formed. The carbon-to-hydrogen ratio of methane is 1:4. EBS has the chemical formula of C38H76. Therefore, the carbon-to-hydrogen ratio of EBS is 1:2. Hence, the breakdown of EBS drops carbon. For this reason, Feldbauer describes the need for a very controlled amount of oxygen to react with the carbon. Moisture is the most controllable form of oxidizing media [7]. The H2O reacts with the dropped carbon, forming hydrogen H2 and carbon monoxide CO, burning off in the sintering atmosphere. The H2O flow rate needs to increase with increased lubricant content and production rates as more carbon will be dropped. However, this is a delicate process and accurate control is critical [7]. If too much H2O injected, then oxidation is the result; if not enough H2O is injected, then carbon will be left behind.
An Innovative Approach
Recently, the development of a new technology is resulting in a paradigm shift of how lubricant removal is being addressed. This new technology is the Vulcan process. It gets its name from the Roman god of steelmaking and uses all the knowledge from the research described above. The Vulcan and its accompanying equipment show it can remove all the lubricant from the compact.
The Vulcan uses lower lubricant removal temperatures to prevent sooting. The optimal temperature range for the removal of lubricants is low in comparison to typical sintering processes. A conventional sintering furnace will have difficulty holding the compacts in this range because of the radiant style of heating used. The result is a rapid heating of the product and lubricant that dissociates to form soot, both internal and external to the compact. This optimal temperature range requires a convective heating source for the initial portion of the Vulcan process. The convective heat transfer coefficient is a strong function of atmosphere chemistry and velocity [1]. This, along with loading and belt speed, provide a great deal of heating control, which enables the time in the optimal temperature range to be adjusted to accommodate the density, product loading, and mass of the compacts.
The Vulcan allows for the manipulation of the atmosphere to rid the particles of barriers. It uses an independent moisture source that provides fine control of the amount of water introduced. The injection location and fine-moisture control provide moisture to react with any carbon dropped during the lubricant break-down. Moisture injection in conventional systems is typically insufficient, difficult to control, or influences the thermal control of the system — all negatively influencing the quality of the lubricant-removal process.
Comparing Performance
To compare the conventional sintering technology to the Vulcan process, FC-0208 powder was paired with the lubricant Acrawax C TM (EBS). Lubricant content of the samples varied from 0.30 wt%, 0.50 wt%, and 0.75 wt%. Density of the samples varied from 6.8 g/cm3, 7.0 g/cm3, and 7.2 g/cm3. The Transverse Rupture Strength (TRS) bars were loaded in two conditions (Figure 1). They were loaded in either Single Stack or Double Stack loading. In Single Stack loading, TRS samples were placed on top of ceramic plates. In Double Stack loading, TRS samples were placed in between ceramic plates; this loading was done to simulate the doubling of production. These loadings were used as worst-case scenarios as they increase thermal mass and hinder interaction with furnace atmosphere. The samples were then sintered either with the conventional process or with the Vulcan process.
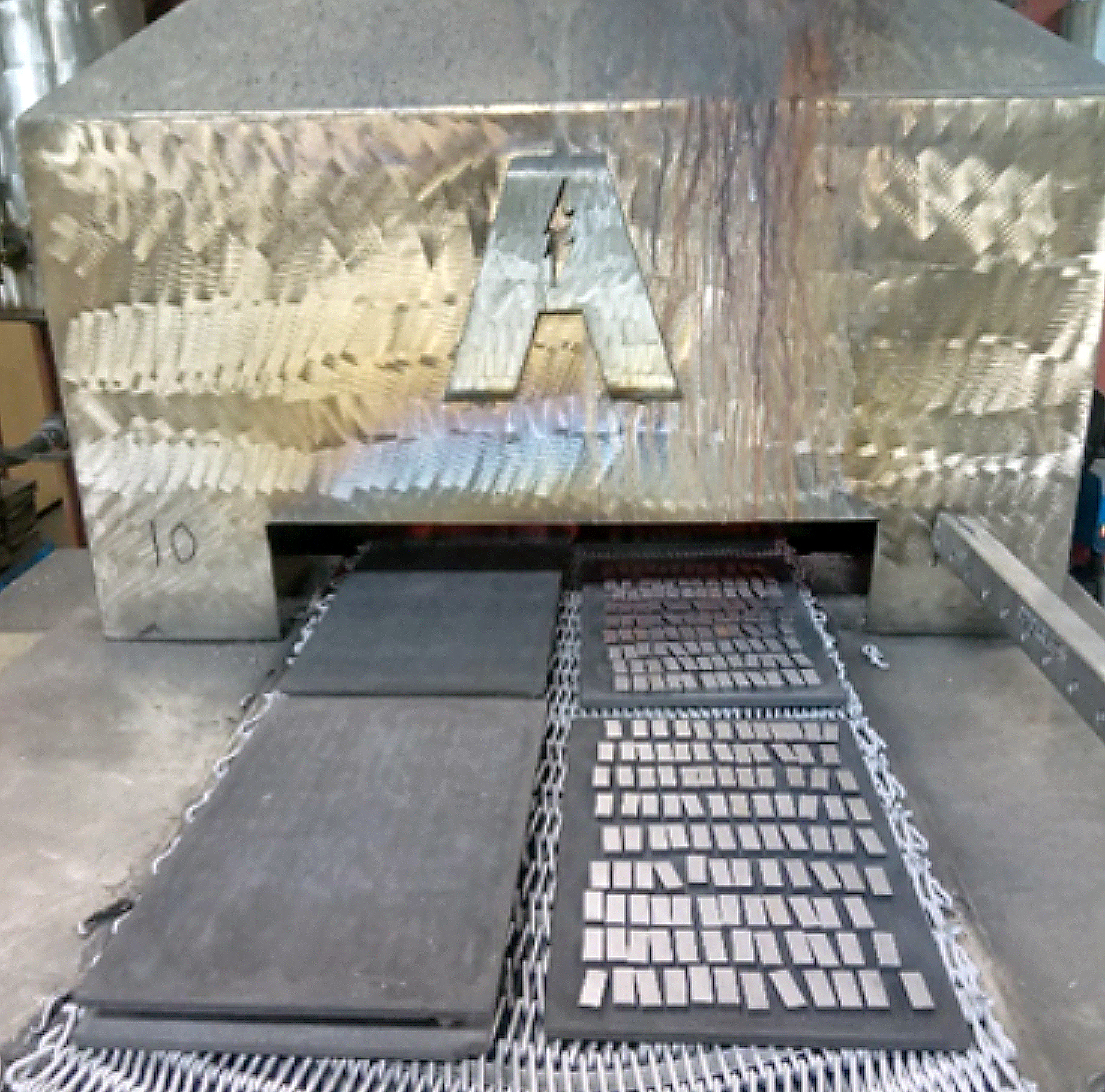
The conventional and Vulcan furnaces each contain eight zones of heating control, four zones in the high-heat section, and four zones in the pre-heat section. Both furnaces have a 240 inch (6.10 m) pre-heat box; both have a 240 inch (6.10 m) high-heat section. The cooling sections in the Vulcan and conventional furnaces are identical. The only difference, in appearance, is that the Vulcan has a 4 foot (1.22 m) Zone 0 that allows the compact to be exposed to hot gases moving forward in the furnace before parts enter the pre-heat box. These zones were set for each furnace to achieve optimal lubricant removal. Both processes were set with the belt speed of 6 in/min (15.2 cm/min), sintering temperature of 2,075 °F (1,135°C), and approximately 22 minutes at the sintering temperature for each system. Note sintering times are the time that the compact is within 50°F (28°C) of the target sintering temperature.
The thermal profiles of the two processes reveal where the paradigm shift lies. The difference allows the part to be in the optimal lubricant temperature range, 350°F to 1,000°F (177°C to 537°C), 2.4 times greater in the Vulcan furnace compared to the conventional furnace.
Weight loss
Weight loss was evaluated from green to sintered. All weight losses were greater than the original amount of lubricant. Recall there are two factors that contribute to weight loss: the removal of the lubricant and the removal of oxygen during the oxide reduction step. However, this type of comparison is still relevant as the amount of oxide in the powder is assumed to be equal, so any difference in weight loss can be attributed to the loss of lubricant.
In all cases, the weight loss was less in the conventional process for all comparable conditions. However, the differences were very small. It is also important to note that weight loss is often better for the Vulcan double stack condition when compared to conventional single stack of comparable conditions.
This difference can be explained by a couple of contributing factors. First, the TRS bars being on the ceramic plates increase thermal mass and slows the heating rate of the samples. With the belt speeds being identical, this causes the time the samples are in the optimal lubricant temperature range to be slightly less in the double stack condition. Second, the loading condition hinders interaction with furnace atmosphere. This hindrance with the sintering atmosphere would slow the reaction with the exiting lubricant. This slowing of the reaction can lessen weight loss by leaving some lubricant/carbon behind.
The weight loss study data showed some differences, but the data was inconclusive on which process was performing better.
Transverse Rupture Strength (TRS)
Transverse Rupture Strength data was collected from each processing condition. The thought was that, with more lubricant removed from the compact, the particles would be in greater contact with one another. This would result in a better-quality sinter and increases in Transverse Rupture Strengths. The data shows that TRS strengths are greater for the Vulcan in all comparable conditions.
Figure 2 is the TRS strength data for the molded density 6.8 g/cm3. The red lines are the conventional data, and the blue lines are the Vulcan data. The lubricant content increases to the right, with the square 0.30 wt%, X 0.50 wt%, and triangle 0.75 wt%. The single stack loading data is grouped on the left and double stacked on the right. The TRS strengths favor the Vulcan by 6.9 to 14.4%. The samples processed in the Single Layer condition in the Vulcan have TRS strengths that vary by less than 0.6%. This shows that, at this molded density and condition, the Vulcan provides little variability in TRS strengths, no matter lubricant content.
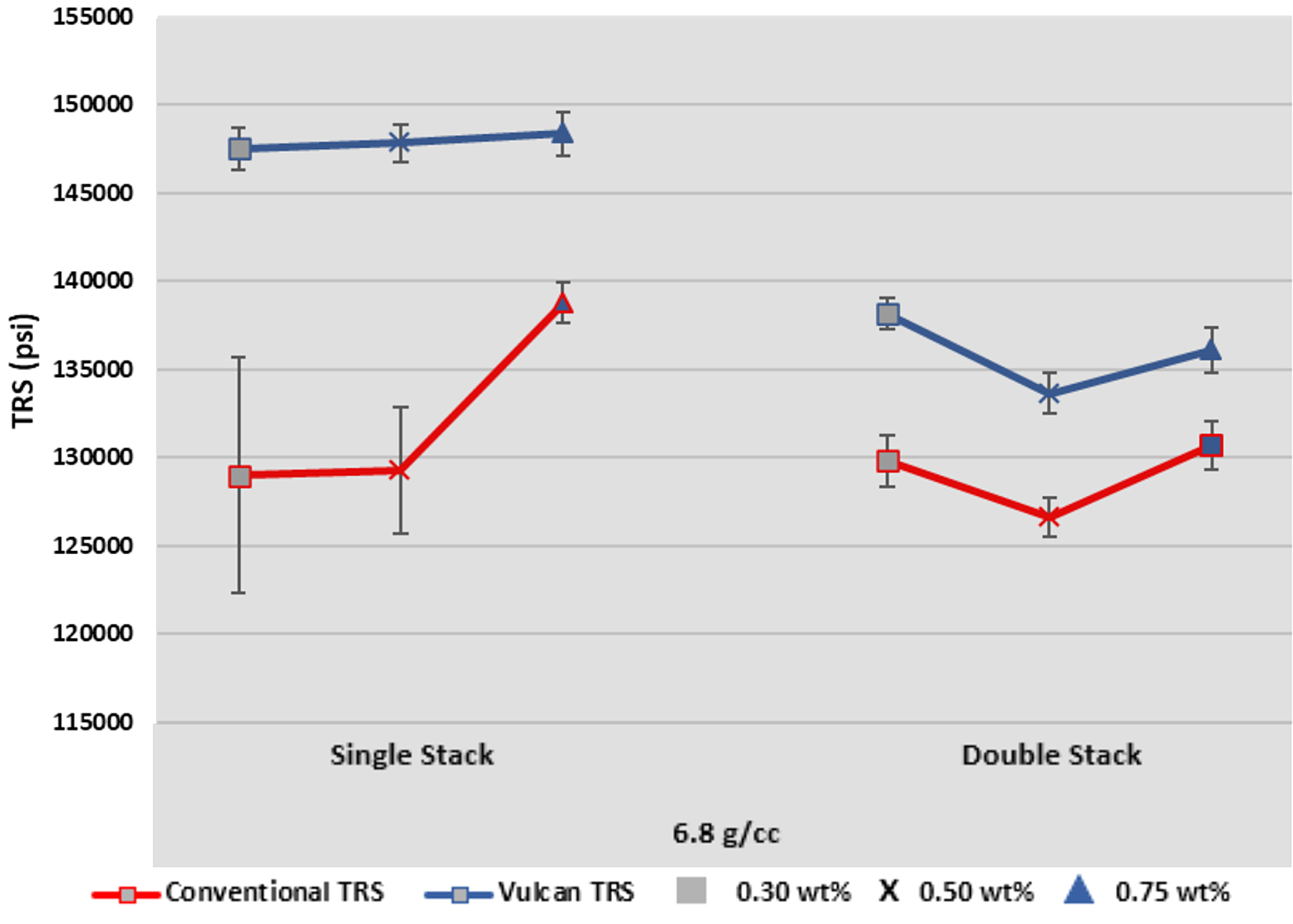
The data from Figure 3 continues to demonstrate the importance of the lubricant removal. As the molded density is increased, there is a significant improvement in strength for those samples sintered in the Vulcan process.

At a molded density of 7.2 g/cm3, a density that is known to be a challenge for the EBS system, the Vulcan shows a large improvement in the properties of the product. This density yields differences in TRS strengths up to 18.0% greater for samples sintered in the Single Layer condition and 3.8% for samples sintered in the Double Stack condition.
Overall, the Vulcan’s TRS strengths are greater for the Single Layer condition by 5.6 to 18.0% and for the Double Stack condition by 1.2 to 6.4%. Therefore, the Vulcan produces better quality parts no matter the lubricant content or density.
In nearly all cases, the product sintered in a double-stacked condition on the Vulcan has strengths equal to or better than those sintered in a single-stacked condition on the conventional system. In the double-stacked condition, the Vulcan has strengths up to 7.1% better than those sintered in a single stacked condition on the conventional system. This would indicate the Vulcan has the potential to produce at twice the production rate and still produce a product equal to or superior to one sintered on a conventional furnace.
Hardness: Rockwell B (HRB)
During the processing of the TRS samples, parts were allowed to normalize. Therefore, any difference seen in hardness can be attributed to lubricant removal increasing the quality of sinter, not from processing. With the lubricant removed, there is no barrier between the particles. With the particles in greater contact with one another, porosity is decreased. The result of decreasing porosity is increasing hardness. The data shows hardness values favor the Vulcan Process for all comparable conditions. The Vulcan increases hardness in the Single Stack condition from 2.4% to 6.3% and in the Double Stack condition up to 5.8%.
Data from Figure 4 shows the largest difference in hardness is seen in the 6.8 g/cm3, 0.50 wt% single layer samples. The HRB hardness favors the Vulcan process by a difference of 6.3%. This can be explained by lubricant removal increasing the quality of the sinter. The 6.8 g/cm3 samples are the most porous of those tested. The more porosity the lower the hardness value. Therefore, a better-quality sinter in a porous sample, results in a larger percent difference in hardness.
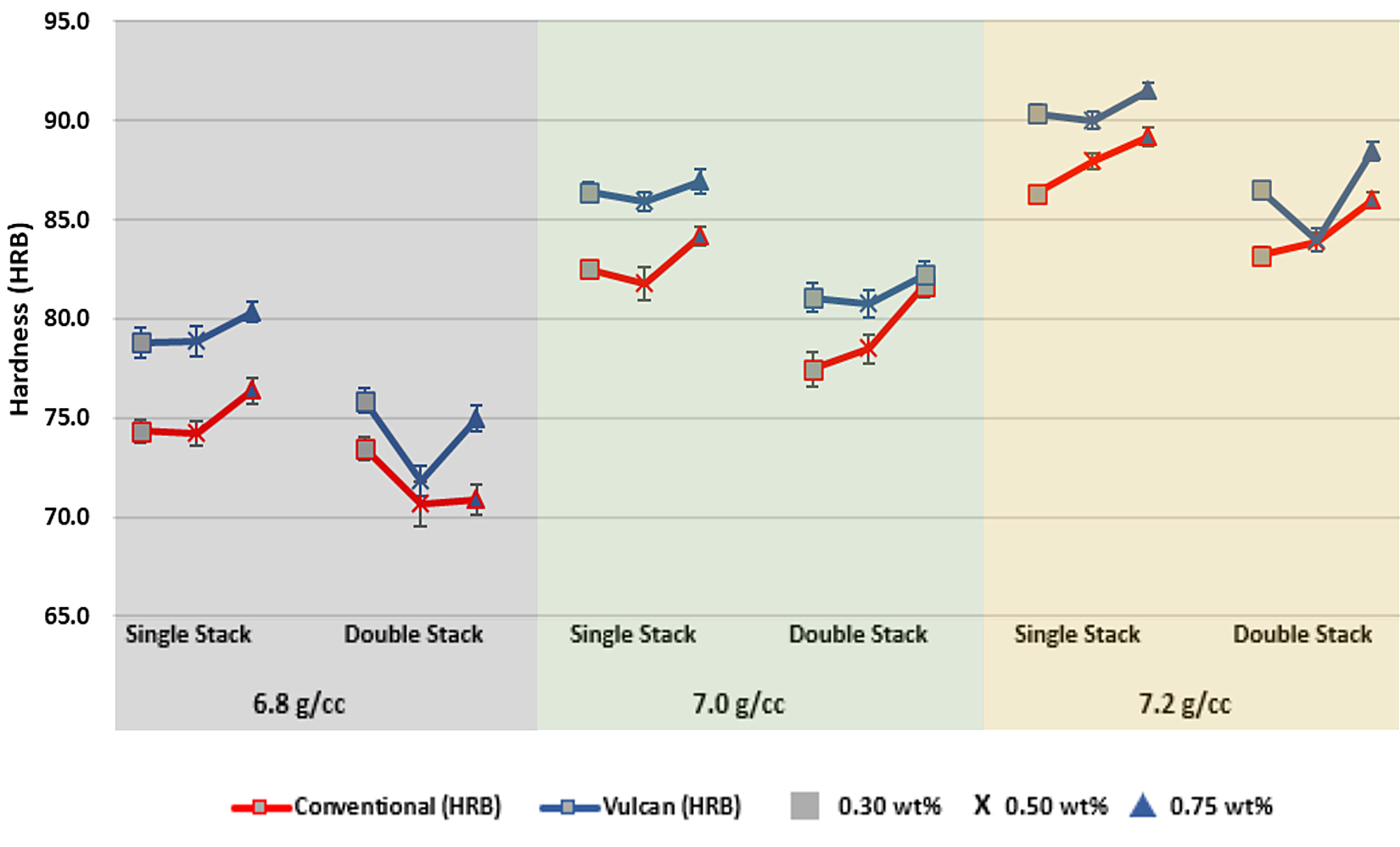
As molded density increases, the samples become less porous. The result of less porosity is an increase in hardness. The data shows the importance of lubricant removal. The Vulcan provides an increase in hardness for all comparable conditions. In the
7.0 g/cm3 samples, the Vulcan increases hardness by 3.2% to 5.1% in the single layer condition and 0.7% to 4.6% in the double stack condition. The Vulcan 7.2 g/cm3 density single stacks samples hardness values are increased by up to 4.7% and by up to 4.0% in the double stack condition.
The data in Figure 4 reveals Vulcan hardness values are greater for all comparable conditions no matter the lubricant content or density. Therefore, the hardness data shows that, by removing more lubricant, the Vulcan provides a better-quality sinter compared to the conventional furnace.
Density
The removal of all the lubricant will allow for a better-quality sinter because the particles will be in greater contact. This greater contact will reduce porosity within the component and, therefore, increase density.
The 6.8 g/cm3 density samples from both processes were evaluated for Archimedes density. The data shows the Vulcan provides more dense parts. In the single-layer condition, the Vulcan increases density by 1.2% and in the double stack condition by 1.7%.
The greater increase in the double-stack condition can be explained by the fact that the conventional furnace struggles with lubricant removal in this loading condition. In nearly all the testing, in all comparable conditions, the conventional double stack saw the lower values. This being lower allows for a greater increase in physical properties.
Scanning Electron Microscope (SEM) Images and Pore Analysis
The Vulcan is providing an increase in all the properties tested. With all the lubricant removed, the particles would be in greater contact. This contact is providing a better-quality sinter.
Figure 5 shows the fracture surfaces of 6.8 g/cm3, 0.50 wt% samples with the conventional sintered sample on the left and the Vulcan process sample on the right. Both scanning electron microscope (SEM) images are at 160X. Note the fracture surface of the sample sintered in the Vulcan is less porous and appears to have more of a shear fracture surface, compared to the more porous facture surface of the conventional sample. Therefore, the Vulcan, by appearance, is providing a better-quality sinter.
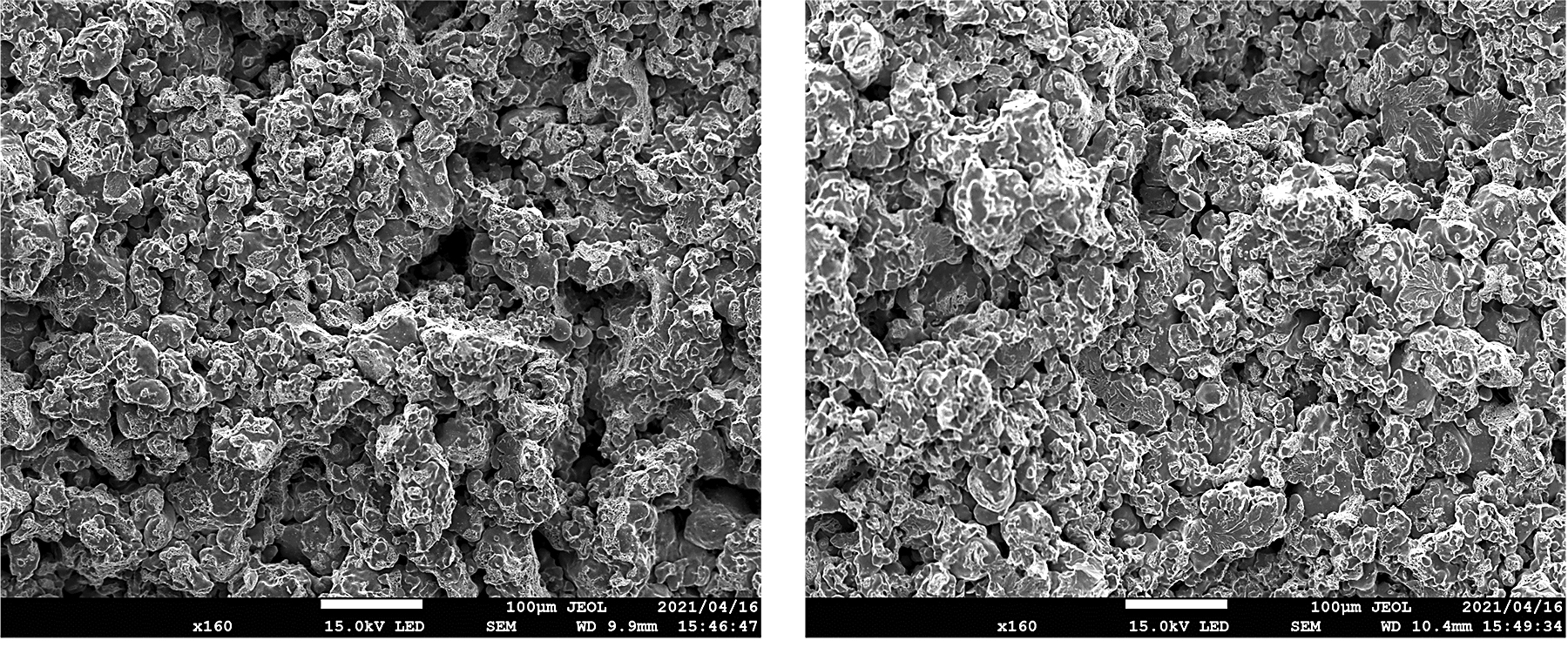
Also, with more lubricant removed, there would be less carbon present within the pores of the compact. FC-0208 powder has a nominal carbon content of 0.6 wt% to 0.9 wt% [3]. Using the SEM, the pores of the fractured 6.8 g/cm3, 0.50 wt% samples were evaluated for what elements were present. The analysis revealed the average pore carbon present in the conventional sample is 10.4 At%. While the Vulcan’s average pore carbon content is 6.4 At%. Therefore, the conventional process leaves 64% more carbon behind in the pores than the Vulcan process does. This difference in carbon content results in TRS strengths increased by 14.4%, hardness improvement of 6.3%, and a 1.2% denser part.
Total Carbon
Total carbon analysis was conducted on the same 6.8 g/cm3, 0.50 wt% samples. This was done to further verify the Vulcan process is removing more lubricant than the conventional process. With the amount of carbon within the powder being consistent, any difference seen in the total amount of carbon can be attributed to lubricant not removed. Therefore, the lesser the amount of carbon present, the more lubricant was removed.
The data shows there is less total carbon in the parts processed in the Vulcan no matter the condition. In the single-layer condition, the Vulcan total carbon was found to be 0.73% compared to the conventional 0.74%. With less carbon in the part, physical properties are increased: TRS strength by 14.4%, hardness by 6.3%, and density by 1.2%.
Likewise, in the double-stack condition, the Vulcan total carbon of 0.73% betters the conventional 0.74%. The lesser amount of carbon in the part increases physical properties: TRS strength by 5.6%, hardness by 4.3%, and density by 1.7%.
Conclusion
A comparison was made of the Vulcan process to a conventional sintering process for an EBS lubricant system at various lubricant contents, densities, and loading conditions. In all cases, the Vulcan demonstrated the importance of removing the lubricant and the resultant improvement on physical properties.
Complete removal of the lubricant greatly improves the properties of a sintered component. A part loaded in a single layer on the Vulcan may have up to an 18.0% better Transverse Rupture Strength, up to 6.3% better Rockwell B hardness, and 1.2% increase in density than one sintered in a single layer on a conventional furnace.
The Vulcan affords two distinct advantages over the conventional sintering process. The producer has a choice in how to realize these advantages. They can produce a superior quality product, or they can produce a comparable quality product at twice the rate.
Special Thanks
The authors would like to extend a special thanks to Symmco Inc. for granting us access to its laboratory. Without Symmco’s cooperation and hospitality, the project would not have been possible.
References
- DeWitt, F. P. (2002). Fundamentals of Heat and Mass Transfer. New York: John Wiley & Sons, Inc.
- Edward Levanduski and Stephen L. Feldbauer, P. (2010). Observations in Lubricant Removal. PowderMet (pp. Presentation, Special Interest Session). Fort Lauderdale, FL: Metal Powder Industry Federation.
- Federation, Metal Powder Industries. (2007, April 16). Material Standards for PM Structural Parts. Princeton, NJ: Metal Powder Industries Federation. Retrieved from MPIF Standard 35.
- Lonza Group. (2011, February 9). Lonza_ProductDataSheets_Acrowax_PDS_29679(3).pdf. Retrieved from http://bio.lonza.com/go/literature/2805.pdf.
- Robert Powell, C. S. (2013). Lubricant Transport within a Powder Metal Compact durnign Pre-Sinter. PowderMet. Chicago, IL: Metal Powder Industry Federation.
- Standard Test Methods for Metal Powders and Powder Metallurgy Products. (2010). Princeton, NJ: Metal Powder Industry Federation.
- Stephen L. Feldbauer, P. (2020, January). A Review of Lubricant Removal Systems and the Latest Technology. Industrial Heating, pp. 24-27.
About the authors
Scot E. Coble and Jacob P. Feldbauer are with The Pennsylvania State University. Amber Tims, PMT, is with the North American Hoganas Co. Craig Stringer, Ph.D., is with Atlas Pressed Metals. Stephen L. Feldbauer, Ph.D. is with Abbott Furnace Company.