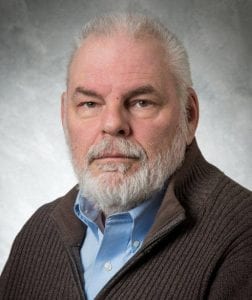
In this column, we will discuss controlling the distortion of aluminum heat-treated components.
The heat treatment of aluminum (solution heat treatment, quenching and aging) are critical processes to ensure that the desired mechanical and corrosion properties are achieved. Of these steps, quenching is perhaps the most critical of all the operations. If quenching is too fast, properties are met, but the part may have excessive distortion or residual stresses. This can result in shortened life due to residual stresses or result in additional non-value-added straightening of the component.
The typical heat treatment for aluminum consists of solution heat treatment to approximately 525°C to ensure that all solute is in solution. Parts are then typically quenched into water or polymer quenchants [1] [2]. Following quenching, parts are then straightened. If parts are unable to be straightened immediately after quenching, the parts are placed into a sub-zero freezer (typically at –28°C) to prevent hardening due to natural aging [3]. Once time is available, the parts are removed from the freezer and allowed to warm to room temperature. Straightening of the parts is then performed [3]. Parts are naturally aged, depending on the alloy and the desired temper. Parts are then artificially aged at an elevated temperature (121°C to 176°C) to the desired final properties and temper.
Quenching is the most critical step in aluminum heat treating. The objective of quenching is to preserve the solid solution formed at the solution heat-treating temperature by rapidly cooling to room temperature. Quenching is a balance of supersaturation and diffusion rate [4]. If quenching is too fast, then properties are achieved, but distortion or warpage of the parts may occur. If quenching is too slow, then excessive grain boundary precipitation can occur [5]. This removes the solute from aging and has detrimental effects on corrosion properties. Generally, the highest strengths and corrosion resistance attainable are those associated with the fastest quenching rates. However, the amount of warpage or distortion that occurs during quenching tends to increase with the rate of cooling. In general, the best quench rate is the slowest quench rate that achieves properties.

Controlling Distortion
Aluminum is extremely prone to distortion. During solution heat treatment, temperatures are used that are very close to the liquidus temperature [6]. This results in very high plasticity and low strength at typical solution heat treating temperatures [7].
In addition to the poor strength at elevated temperatures, aluminum also has a large coefficient of linear expansion. This results in large growth of aluminum during solution heat treatment and contraction during quenching. If the part is constrained, then high strains and stresses are developed in the part. If these stresses exceed the yield strength at temperature, then permanent set of the part could occur, resulting in distortion of the part. This indicates that racking and constraint of the parts are important to control the distortion of the part.
Residual Stresses Prior to Heat Treatment
Aluminum parts are typically forged, cast, or formed prior to heat treatment. Each of these processes produces significant tensile or residual stresses [8] [9] [10]. Machining of these forgings or castings can also produce significant residual stresses [11] [12]. The use of a stress relief process after major manufacturing steps will also reduce distortion in heat treatment.
Solution Heat Treatment
With excessive heat-up rates, thermal gradients can be developed in the part that can exceed the yield stress at temperature [13]. This is especially true when there are very thick and very thin portions of the part. The thin sections will heat rapidly, while the larger sections will lag in temperature behind the thin sections. If the thermal gradient is high enough, distortion of the part can occur. If residual stresses are present in the part from prior operations, then distortion can occur from relief of those stresses.
Immersion Rate
The rate of immersion during quenching plays an important role
in reducing the distortion of quenched aluminum. Aluminum parts should be immersed into the quench rapidly. This immersion rate is often confused with the quench delay; however, the immersion rate is the velocity at which the parts enter the quenchant. While
it is sometimes not possible to adjust this rate due to furnace
design, it is often a variable that is overlooked. The immersion rate should be on the order of 0.15 m/s to 3 m/s [14]. This is shown in Figure 1.

Quenchant
Of all the possible “defects” occurring during the heat treatment of aluminum, distortion during quenching is the most common. It is probably responsible for most of the non-value-added work (straightening) and costs associated with aluminum heat treating. An extreme case of distortion is shown in Figure 2 [6].

Water is the most common quenchant for all aluminum alloys. It is easy and inexpensive to obtain, and it is readily disposed unless severely contaminated. Water is used as a quenchant from ambient temperatures for sheet metal, to 90°C for castings and thick forgings to reduce quenching thermal stresses.
As the temperature of water is raised, the stability of the vapor phase increases, and the onset of nucleate boiling in a stagnant fluid is suppressed. The maximum rate of cooling is decreased, and the overall rate of cooling is also decreased. This can result in non-uniform quenching of part, with resultant distortion and high residual stresses.
Polyalkylene glycol quenchants (PAG) were developed to provide a quench rate in between that of water and oil. By control of agitation, temperature, and concentration, quench rates like water can be achieved. PAG quenchants are described by AMS 3025 [15], and the concentration is governed by AMS 2770 [16] for aerospace alloys. The benefits of quenching in a PAG are shown in Figure 3.

Part Orientation and Racking
Racking of the parts is critical [17]. The parts should be fully supported, with the loads spread out over a large area, since the creep strength of aluminum is poor. Parts, particularly sheet metal parts, are often fixed into place with steel or stainless wire to hold the parts. This practice can increase distortion or cause damage to the part. Aluminum has a very high coefficient of thermal expansion, while steel has a coefficient of thermal expansion that is approximately half that of aluminum (13 x 10-6 mm/mm-°C for aluminum and 8 x 10-6 mm/mm-°C for carbon steel). This means that the aluminum parts will grow twice as much as the steel constraining wires. Aluminum parts should be wired loosely and allowed to freely expand without constraint.
Conclusions
In this very brief article, the causes of distortion when heat treating aluminum alloys are discussed. This is a very complex subject, and many papers have been written to cure the very expensive problem of aluminum distortion during heat treatment.
Should you have any questions or comments on this column, or suggestions for further articles, please contact the author or editor.
References
- ASTM, “Standard Practice for Heat Treatment of Wrought Aluminum Alloys,” ASTM International, West Conshohocken, PA, 2017.
- ASTM, “Standard Practice for Heat Treatment of Aluminum-Alloy Castings from All Processes,” ASTM International, West Conshohocken, PA, 2017.
- Boeing, “Heat Treatment of Aluminum Alloys,” 2017.
- J. E. Hatch, Aluminum: Properties and Physical Metallurgy, Metals Park, OH: American Society for Metals, 1984.
- D. S. MacKenzie, “Effect of Quench Rate on the PFZ Width in 7XXX Aluminum Alloys,” in Proceedings from 1st International Symposium on Metallurgical Modeling for Aluminum Alloys, 13-15 October, 2003, Pittsburgh, PA, 2003.
- D. S. MacKenzie, “Heat Treating Aluminum for Aerospace Applications,” in Proc. 1st ASM Int. Surface Engineering and 13th IFHTSE Congress, Columbus, OH, 07-10 October 2002.
- Department of Defense, Metallic Materials and Elements for Aerospace Vehicle Structures, Department for Defense Handbook, 2003.
- M. Prime and A. T. DeWald, “The Contour Method,” in Practical Residual Stress Measurement, G. S. Schajer, Ed., Hoboken, NJ, Wiley, 2013, pp. 109-138.
- D. Hornbach and P. Prevey, “Development of Machining Procedures to Minimize Distortion During Manufacture,” in Proc. ASM Materials Week, Indianapolis, IN, 1997.
- G. W. Kuhlman, “Forging of Aluminum Alloys,” in ASM Handbook: Metalworking – Bulk Forming, vol. 14A, S. L. Semiatin, Ed., Metals Park, OH: ASM International, 2005, pp. 299-312.
- B. Denkena and L. de Leon, “Machining Induced Residual Stress in Wrought Aluminum Parts,” in Proc. 2nd Int Conf. Distortion Engineering, IDE 2008, Bremen, Germany, 17-19 September, 2008.
- B. Denkena, D. Boehnke and L. de Leon, “Machining induced residual stress in structural aluminum parts,” Production Engineering, vol. 2, no. 3, pp. 247-253, 2008.
- A. Deschamps and Y. Brechet, “Influence of Quench and Heating Rates on the Aging Response of an Al-Zn-Mg-(Zr) Alloy,” Mat. Sci. Eng., Vols. 217-222, pp. 43-56, 1998.
- D.S. MacKenzie, “Quenching of Aluminum Alloys,” in Heat Treating of Nonferrous Alloys, Metals Handbook 4A, vol. 4E, G. E. Totten and D. S. MacKenzie, Eds., Materials Park, OH: ASM International, 2016.
- SAE, “AMS 3025E Polyalkalene Glycol Heat Treat Quenchant,” SAE, Warren, PA, 2018.
- SAE International, “Heat Treatment of Wrought Aluminum Alloy Parts,” SAE International, Warrendale, 2015.
- D. S. MacKenzie, “Metallurgical Aspects of Distortion and Residual Stresses in Heat Treated Parts,” in 23rd IFHTSE Heat Treatment and Surface Engineering Congress, April 18-21, Savannah, GA, 2016.
- J. W. Evancho and J. T. Staley, “Kinetics of Precipitation in Aluminum Alloys During Continuous Cooling,” Met. Trans., vol. 5, pp. 43-47, January 1974.