Editor’s note » This is part one of a three-part series on carburized steel mechanical properties.
Carburizing is a mature heat-treating process commonly used for gears. The carbon content of the surface is typically increased to about 0.70 to 1.00 percent, and the part is oil quenched. This provides a hard, wear resistant surface or case, and a softer more ductile core. The typical surface hardness after quenching is about 65 HRC and 62 HRC after tempering at 177°C. It is a robust process that is tolerant of many processing variables. It provides good bending and contact strength as well as good bending and contact fatigue life. It is commonly believed the case has a high tensile strength with limited ductility, while the core can range from moderately high to low strength depending on the application. In bending as well as in torsion, the applied stress is maximum at the surface, so placing the highest strength at the surface is ideal.
Modeling carburized gears
Carburized gears can be modeled by using axial strain-based static and tension compression data for the case and for the core. Based on SAE J413, the typical carburized case of about 62 HRC should have a tensile strength above 2,269 MPa, while a typical core at 30 HRC would be about 952 MPa. Around 1998, AISI (American Iron and Steel Institute) began a joint steel producer and user program to develop axial strain-based fatigue and static data for carburized, through-hardened, normalized, and induction-hardened steels. The Bar Fatigue Committee was active for about 20 years and, at one point, came under the umbrella of SMDI (Steel Market Development Institute). The data now resides at the University of Waterloo [1].
Most of the data was generated using 5.08 mm diameter, 10.8 mm gauge length test bars. The through-carburized bars representing the carburized case were typically carburized for 24 to 26 hours at 927°C. This cycle didn’t completely through-carburize the sample but provided a core that was just a few points HRC lower than the surface. It was later determined that 36 hours was necessary to completely through-carburize this diameter so the hardness was uniform though the complete cross section. Obviously, these carburizing cycles are much longer than those used for most automotive and truck gears, but they are necessary to through-harden the test bar cross section.
Figure 1 is a chart showing ultimate strength vs. hardness for the data through the first 141 data sets in the database.
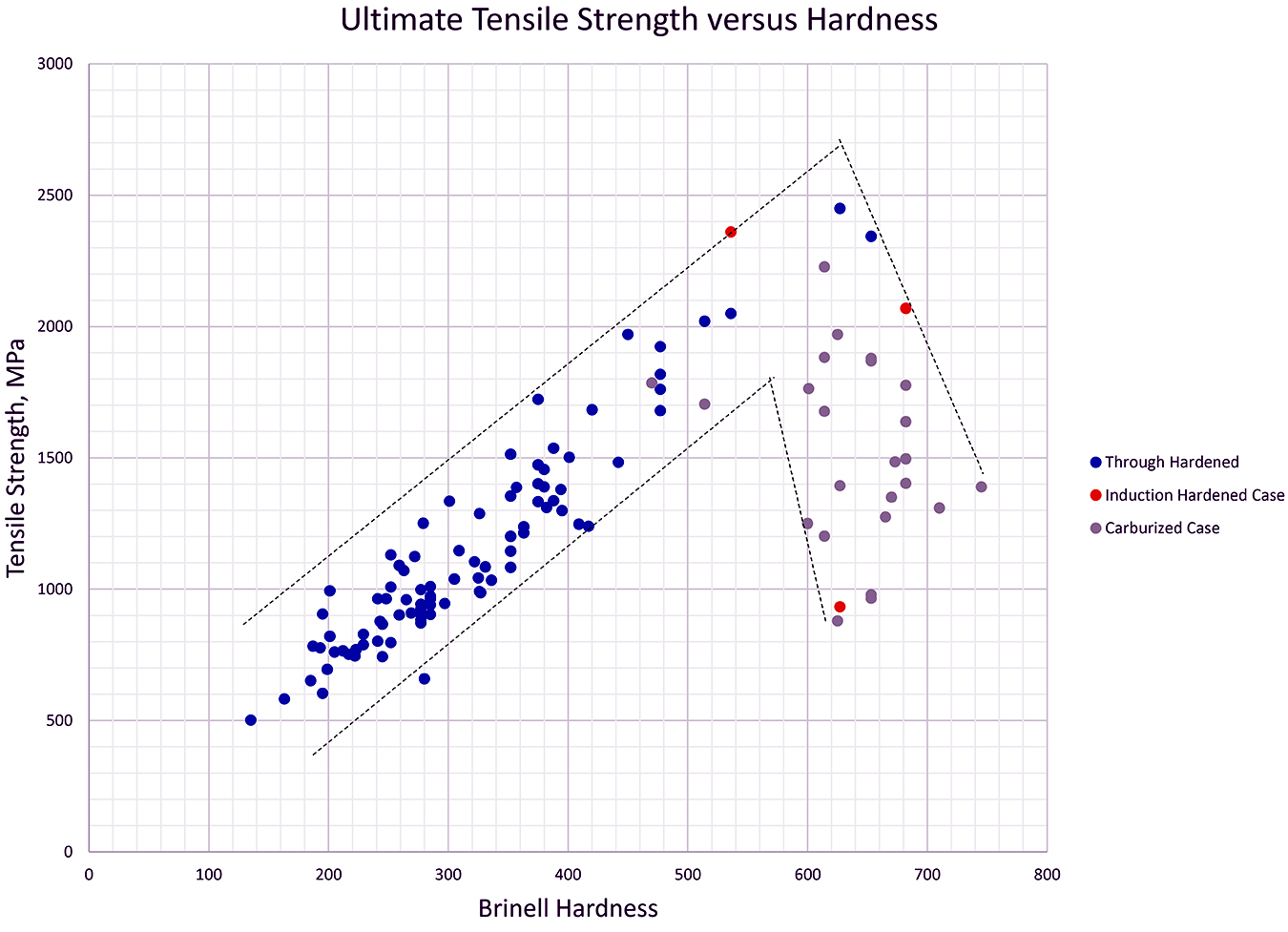
This includes quench and tempered through-hardened samples, carburized case and core samples, normalized samples, and induction-hardened samples. There is a good correlation between hardness and strength up to about 575 Brinell or 56 HRC. Above this hardness, the correlation ends, and the strength may continue to increase, or it may significantly decrease. The two through-hardened samples that did increase to 2,343 and 2,450 MPa were 5160 and 9254 spring steel, respectively. Their hardness was 58 to 60 HRC, and the elongation was 0.0 and 3.9 percent respectively. The induction-hardened sample in the same area was 1050M steel induction-hardened and tempered to 54 HRC. The tensile strength was 2,360 MPa, and the elongation was 16.7 percent. The bulk of the high hardness samples are through-carburized case samples, and they vary between 900 to 2,200 MPa, and the elongation ranged from 0.0 to 12.5 percent. The high end of this strength range is close to where they should be per SAE J413 based on hardness, while the low end is equal to the strength of the core at a hardness of 28 HRC. Obviously, this is too much variation to be useful for design purposes.
Variation study
Around 2013, a study was initiated to determine why there was so much variation [2]; 8620 test bars from five steel sources were machined and polished to an Ra of 0.2 microns and distributed to three member companies for heat treatment. They were carburized at 927°C on a 12.5-hour cycle at source A, a 24-hour cycle at source B, a 36-hour cycle at source A, and a 48-hour cycle at source C. The data is shown in Table 1.
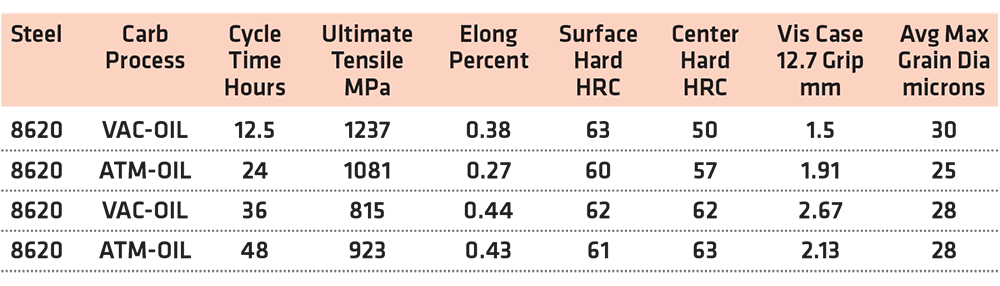
The 12.5- and 36-hour cycles were done in a vacuum furnace with oil quenching, and the other two cycles were done in atmosphere furnaces with oil quenching. Tempering was done at 177°C. No additional hard finishing or polishing was done after heat treatment. After heat treatment, the Ra surface finish on the atmosphere furnace samples was about 0.28 microns, while the vacuum furnace samples increased to about 0.66 microns. Vacuum carburizing produced an etched appearance on the surface and the individual grains were visible. The average tensile strength was 1,237 MPa for the 12.5-hour cycle, 1,081 MPa for the 24-hour cycle, 815 MPa for the 36-hour cycle, and 923 MPa for the 48-hour cycle. The elongation for all samples was less than 1.0 percent, and there was no measurable yield as the deformation was primarily elastic. Table 1 shows the total visual case depth in the 12.7 mm sample grip area after annealing and furnace cooling. Figure 2 shows there is a good correlation between the visual case depth and the ultimate tensile strength. With less carburizing time and case depth, the strength increased, but it is still only about half of what it is projected to be based on hardness.
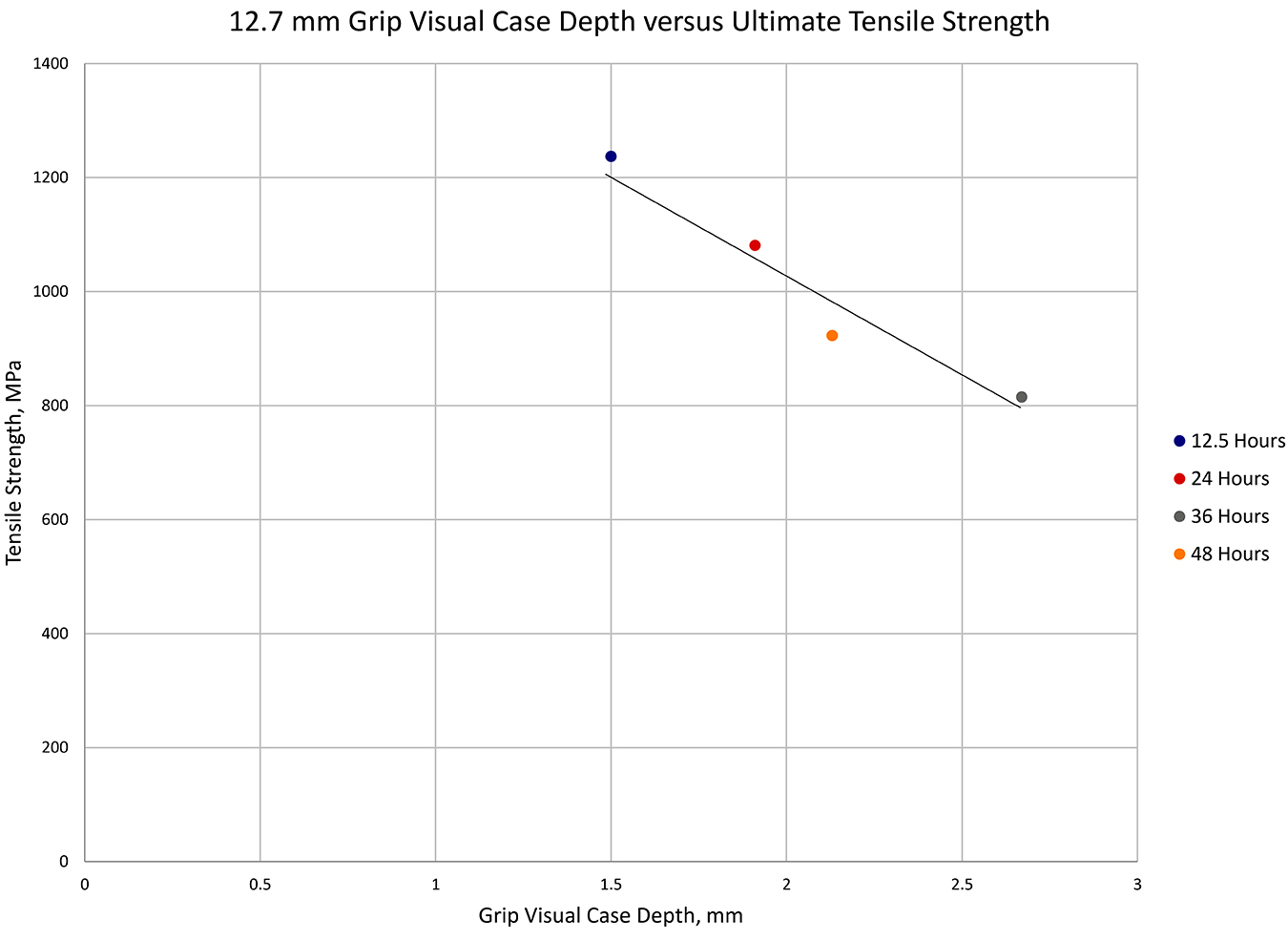
Cycle results
The deepest case depth was actually produced by the 36-hour cycle, and it was uniform through the entire cross section. The 48-hour cycle was reportedly run with a constant carbon potential rather than a boost diffuse cycle, which may explain the lower-than-expected visual case depth. Table 1 also shows the average maximum grain diameter found at the fracture origin on the SEM images for all samples from each heat-treat group. Maximum grain size was measured rather than the average as this represents the worst case. This can be critical in some cases, and it is much easier to determine. The fracture origins were at the surface and were intergranular, and the grain size was relatively constant among the groups. A finer grain diameter would be expected to result in a higher tensile strength [3]; however, the 12.5-hour cycle had the largest grain diameter and the highest strength. The dominant factor for determining tensile strength was the carburized case depth and less was better. Figure 3 shows the maximum grain diameter vs. tensile strength for all samples.
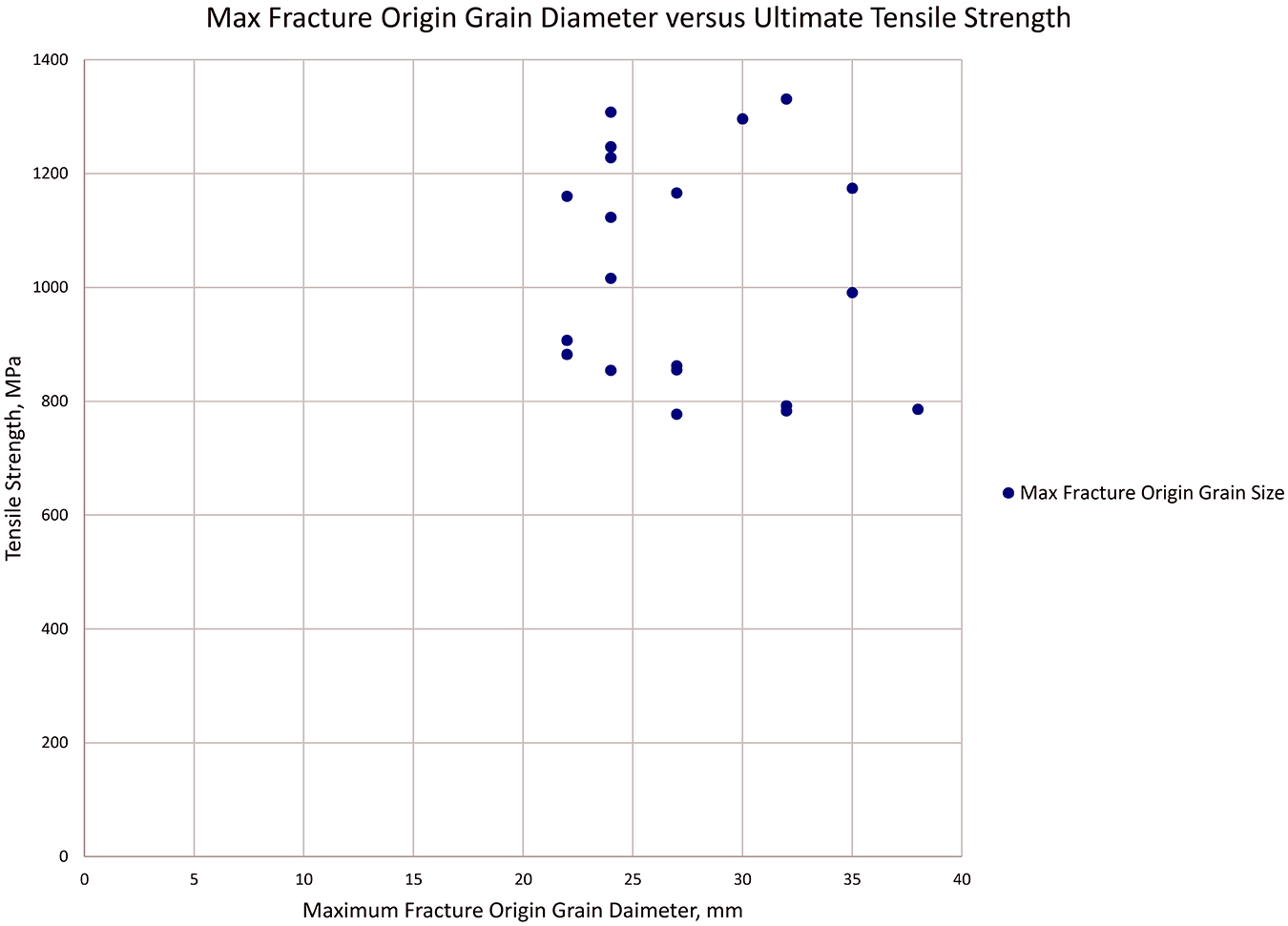

It appears to show no correlation between grain diameter and tensile strength within the range found. However, if we look at the average maximum grain diameter vs. tensile strength for the five different steel sources in Figure 4, we can see a slightly different story. Here there appears to be some correlation between the maximum grain diameter and tensile strength. The steel source or potentially the heat of steel from the same source can also make a difference in the tensile strength.
Additional work was done with 8620 through-carburized case samples and 52100 quench and tempered samples. The 52100 grade is essentially equivalent to the carburized case on 20MnCr5 steel. The 8620 bars were vacuum carburized and oil quenched on the 36-hour cycle, and the 52100 bars were vacuum oil quenched. The bars were tempered at 177°C, 232°C, and 260°C to investigate the effect of tempering temperature. During the AISI/SMDI program, bars were sometimes tempered above 177°C as the surface hardness aim was 58-60 HRC. All bars were machined prior to heat treatment, and there was no hard finishing or polishing before or after heat treatment. The results are shown in Table 2.
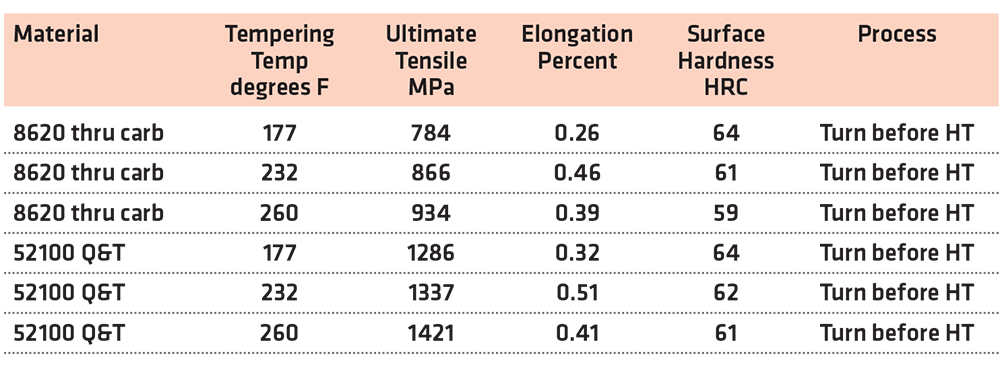
The 8620 sample tempered at 177°C was at 784 MPa, which is the about the same tensile strength as the 8620 samples in the above study. The lack of polishing prior to heat treatment had no detrimental effect on the strength. The strength gradually increased with the tempering temperature to 934 MPa at 260°C, and the surface hardness was still in specification at 59 HRC. The 52100 sample tempered at 177°C started at 1,286 MPa and gradually increased to 1,420 MPa at 260°C. The major microstructural difference between the 8620 and 52100 samples was the grain size. The maximum grain diameter of the 8620 samples was about 50 microns while the 52100 was about 25 microns. This is a potential contributor to the strength difference between the 8620 and 52100 steels [3].
The next step was to through-carburize oversized 8620 bars and hard turn and polish after heat treatment. The results are shown in Table 3.

AISI/SMDI program
During the AISI/SMDI program, bars were sometimes hard finished and/or polished after heat treatment, and this was not always documented. The tensile strength with the 177°C temper was 1,178 MPa, and the strength with the 232°C temper was 1,559 MPa. The hardness for both conditions was still above 58 HRC.
This same exercise was repeated with the 52100 quench and tempered samples. The results are shown in Table 4.
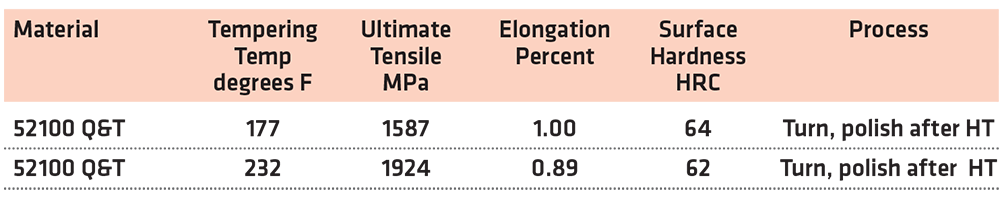
The tensile strength with the 177°C temper was 1,597 MPa, and the strength with the 232°C temper was 1,924 MPa. It is likely tempering at 260°C would come close to the 2,269 MPa target. Hard finishing and polishing after heat treatment coupled with tempering at an elevated temperature is capable of significantly increasing the strength. The key is reducing the surface finish after heat treatment. It would be tempting to blame this on intergranular oxidation (IGO); however, these samples were all vacuum heat treated, and there was no IGO.
Northrop Aircraft study
In 1960, a study was done by Northrop Aircraft titled “Heat Treatment of SAE 52100 Steel” [4]. The tensile strength of oil quench and tempered 52100 austenitized at 829°C and double tempered at 204°C ranged from 1,439 to 2,146 MPa. The elongation of all samples was 0 percent, and the hardness was 60 HRC. The tensile strength of oil quench and tempered 52100 austenitized at 982°C and double tempered at 204°C with and intermediate subzero treatment was 905–907 MPa. Again, the elongation was 0 percent, and the hardness was 61-62 HRC. Tempering at an elevated temperatures of 427°C was able to increase the tensile strength to 1,807 MPa and the elongation to 8.0 percent, but the hardness decreased to 52.5 HRC. This data seems to confirm the strength values found in our investigation, and it also shows the austenitizing temperature can be important.
Is the true strength of the carburized case around 900–1,300 MPa or is it 2,300–2,400 MPa as the hardness would predict? If the steel is not hard finished and/or polished after heat treatment and it is not tempered at an elevated temperature, the answer is 900-1,300 MPa. When samples representing the carburized case are heat treated and the strength is roughly half of what it is supposed to be, it is easy to believe something must have gone wrong. However, the data shows the lower strength is correct. Medium- and high-carbon steels heat treated to higher hardness levels are subject to quench embrittlement [5]. This is normal and not an anomaly. There are ways to cajole the samples to get them to the published values, but this does not represent what is done to actual parts.
If the carburized case is weaker than predicted, how is it possible for carburizing to provide adequate bending strength in order to function as a gear? This will be addressed in part 2.
References
- https://fde.waterloo.ca, Material Fatigue Curves and Calculations, AISI Bar Fatigue Data Base.
- Unpublished work done by G. Fett Dana Corp. between 2013-2015, presented to the AISI Bar Fatigue Committee in 2015.
- J. Wise, D. Matlock, “Bending Fatigue of Carburized Steels: A Statistical Analysis of Process and Microstructural Parameters,” SAE Technical Paper 2000-01-0611, 2000.
- F. Kahlbaugh, Heat Treatment of SAE 52100 Steel, Northrop Aircraft Inc., Unclassified AD 270 415 Armed Services Technical Information Agency, 1960.
- J. Wong, D. Matlock, G. Krauss, “Effects of Induction Tempering on Microstructure, Properties and Fracture of Hardened Carbon Steels,” 43rd Mechanical Working and Steel Processing Conference, ISS, 2001.