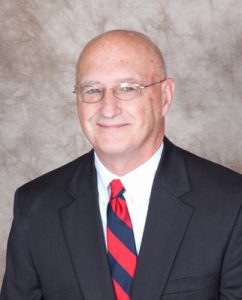
It’s no secret that the heat treating industry is one of the major recipients of the impact when the U.S. Environmental Protection Agency (EPA) makes a regulatory statute. The quantity of natural gas for heating and generating electricity consumed by heat treating is very large. Thus, the movement to reduce NOx (oxides of nitrogen) and other so-called greenhouse gases is of concern for the industry. But what about CO2? Natural gas (methane) is the cleanest burning fuel besides hydrogen, liberating only CO2 and water vapor from its combustion.
Natural gas
It is a myth that green plants and trees convert CO2 into oxygen, but they do consume CO2. In simple terms, oxygen is produced from green plants and trees as the result of the reaction of water vapor (H2O) and daylight during photosynthesis. Also, CO2 reacts to form sugars within the plants. So, both water vapor and CO2 produced by the combustion of natural gas are not a detriment but are helpful to a healthy atmosphere. It has been said that if hydrogen today replaced all of the natural gas burned and totally eliminated all of the CO2 entering the atmosphere, that CO2 dissolved in the oceans and lakes would reenter the atmosphere to regain natural equilibrium.
Heat treating furnaces in the U.S. are heated primarily using natural gas and, in certain locations, using electricity. Table 1 shows the energy distribution for electricity generated by various sources in 2014 and 2015. In 2014, 66 percent of the U.S. electrical energy was produced by hydrocarbon and petroleum products, not counting the natural gas consumed for heating.
Oil quenching
By its organic association, oil will continue to be a reliable quench media for years to come simply because it is applicable to the widest range of steel hardenability. But the discussion about distortion resulting from oil quenching after carburizing continues into the 21st century. The following example addresses that.
AFC-Holcroft’s 36 in. x 48 in. x 36 in. UBQ gas-fired, integral-quench batch furnace with a 3,500-pound maximum payload has a connected natural gas capacity of 1,200 ft3/h. Heating a 3,500-pound charge to 1,700°F requires 770,125 Btu. Non-recuperated radiant tube gas-fired furnaces typically have an efficiency of 50 percent, meaning that half of the gas burned in the radiant tube will leave the tube as exhaust gases. Thus, if 300 ft3 of gas is burned, only 150 ft3 is available to heat the load and overcome furnace wall heat losses. Recovering some of the heat exiting the exhaust by preheating the combustion air can save energy by improving the efficiency to about 63 percent.
Industrial burners are set up to produce 3 percent excess oxygen in the radiant tube exhaust to reduce the potential to create CO. Ideal, or stoichiometric (on ratio), combustion for 1,000 Btu natural gas is an air-to-gas ratio of 10:1. Because 78 percent of air is nitrogen plus 1 percent argon, neither of which contributes to combustion but are heated, 3,000 ft3 of air is required to burn 300 ft3 of natural gas. When the combustion air is preheated to approximately 800°F by passing the air through an exhaust heat exchanger (also called a recuperator), the air/gas combustion efficiency improves. More efficient burner systems are being developed continually.
Why LPC and HPFQ?
Three reasons why the automotive industry adopted low pressure carburizing (LPC) and high pressure gas quenching (HPGQ) include:
- LPC is cleaner than endo gas, it is not explosive, and there is no significant effluent
- It integrates fairly well for a heat-treat system into the manufacturing chain
- HPGQ reduces distortion in gears
However, disadvantages of the process include:
- Electric heating is expensive and still consumes coal
- HPGQ requires high hp motors
- Capital outlay is high
- Maintenance costs are high
- HPGQ with 20 bar helium under the best circumstances cannot match the core hardness achievable using high-speed quench oil
LPC evolved to where it is today primarily because of acetylene, and control technology has matured as well. For example, modeling programs predict the time, temperature, and acetylene flow to achieve a specific case/carbon profile. However, the maximum hardness of that profile can only be realized using high-speed quench oil.

Vacuum furnace design basically remains unchanged, incorporating graphite-based insulation, low-voltage, high-current graphite heating elements, and intricate element-isolation systems. It appears that all LPC systems offered today follow the design intended for high-temperature vacuum furnaces originally developed for processes such as brazing and hardening tool and die steels. Even at elevated temperatures, carburizing will not likely exceed 1,900°F in the foreseeable future.
LPC can be described as a vacuum purge followed by a pressure or flow pulse carburizing process. The hot zone does not need to look much different than a traditional endo-carburizing hot zone with accommodation for the low-pressure vacuum purge. All of the intricacies now associated with vacuum furnaces can be eliminated. Heating elements, electrical isolation, and related maintenance could be a thing of the past. Heating can be done using electric elements or natural-gas radiant tubes made of traditional materials. Insulation can be ceramic, so carbon can be burned out just as in today’s endo-carburizing furnaces. As for quenching, the entire two-chamber system can be designed for vacuum pressures, but not the extremely low levels associated with traditional vacuum furnaces.
The development of the multichamber vacuum furnace could be tied to the decade between 1975 and 1985. Major vacuum furnace suppliers developed their own versions of two- and three-chamber furnaces. Most three-chamber systems consisted of a gas quench at one end and an oil quench at the other with a graphite-lined vacuum hot zone in the center. Some furnaces had an oil quench and loading vestibule in lieu of a gas quench and operated as a straight-through system. LPC, or vacuum carburizing, played a minor role in the process in early multichamber furnaces, as they were primarily used for air hardening and high-speed steels in the attempt to displace salt-bath heat treating. Hot zones were designed to operate as high as 2,400°F. In all cases, the oil quench chamber was evacuated to remove oxygen from the quench oil and purge the air prior to moving the load to the graphite hot zone, which was continuously kept hot. After 1985, multichamber vacuum furnaces began to lose their popularity due primarily to high cost and the beginning of the demand for six-bar HPGQ to accommodate new hotwork die steels.
Marquenching is the go-to process to control distortion in oil quenching. The process involves quenching into oil where the oil temperature is held at just above the Ms (martensite start point) of the alloy. Parts are held in the oil until their core reaches the oil temperature, after which martensite transforms uniformly during post wash and cooling. The oil has a maximum operating temperature, and with atmosphere furnaces, the major issue with oil quality is sludge caused by oil oxidation. This is not an issue with AFC-Holcroft’s LPC/UBQ, because oxygen is precluded from being present above the oil. The advantage of marquenching is emphasized in Figure 1, Figure 2, and Figure 3, which show how surface carbon affects the Ms point for 1320 and 1321 manganese steels [1]. The Ms point decreases with increasing carbon potential.
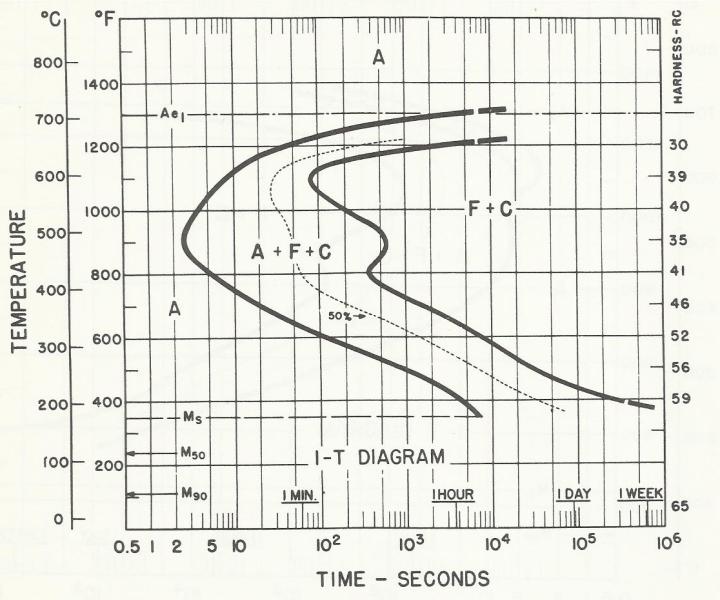
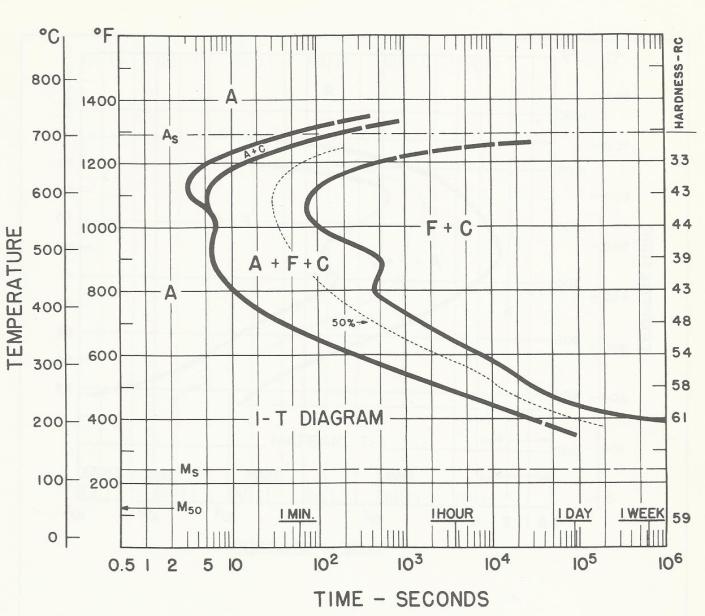

As for LPC, when using a furnace that incorporates a ceramic-lined hot zone and has no cold spots (as found in existing vacuum furnaces), all of the acetylene is available for carburizing, which improves efficiency and reduces the need to account for the surface area of the workload.
Conclusion
Based on these observations, it makes sense for the next generation of carburizing systems to go back to integrating the advances of LPC and the wide application range of modern marquench oils.
Reference
- J. F. Boyce and R. Grange, “Transformation of Austenite in Carburized 1320 and 1321 Steels,” (unpublished USS Research Laboratory report).