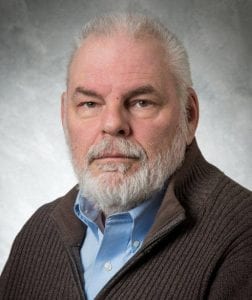
In previous articles, we discussed the role of alloying elements and discussed the solution heat treatment of aluminum. We showed that it is important for all the alloying elements to be in solid solution prior to quenching.
In this article, we will show the most critical aspects of quenching aluminum. In many respects, this is the most critical operation in the entire process of heat-treating aluminum.

Quenching
The fundamental objective of quenching is to preserve, as nearly as possible, a solid solution formed at the solution heat-treating temperature by rapidly cooling to room temperature. This maintains the solute in a super-saturated solid solution and maintaining an adequate supply of vacancies required for subsequent precipitation. When quenching rates from the solution temperature are not sufficiently rapid to retain the solute in solution or maintain adequate vacancy concentration, the solute will precipitate on grain boundaries, or dispersoids. Vacancies migrate very rapidly to disordered regions like grain boundaries. The amount of precipitation occurring during quenching reduces the amount of subsequent hardening possible. This is because as solute is precipitated from solution during quenching, it is unavailable for any further precipitation reactions. This results in lower tensile strength, yield strength, ductility, and fracture toughness.
The fastest quenching rate will achieve the best combination of strength and toughness. Resistance to general corrosion and stress-corrosion cracking is also improved with maximum quenching rates. The preferential formation of precipitated phases at grain boundaries during quenching or subsequent artificial aging also is extremely important in relation to corrosion resistance and stress corrosion cracking. Grain boundary precipitation is frequently accompanied by development of thin layers of precipitate-free zones adjacent to the boundaries. (Figure 1) Resistance to corrosion and to stress-corrosion cracking are generally improved by maximum rapidity of quenching, although some of the alloys used in artificially aged tempers are exceptions to this rule. For example, the resistance to corrosion of aluminum-copper alloys in the artificially aged condition is less dependent on a rapid quench than when in the naturally aged temper.

A maximum cooling rate is not the only thing that must be considered. Residual stresses and distortion increase with increasing quench rates. A balance between the maximum quench rate and distortion must be maintained. In general, this means that the fast quench rate that just satisfies the desired properties should be used.
The quench sensitivity of the alloy means that some alloys are less sensitive to quench rate than others. For example, an extended delay in quenching generally results in lower mechanical properties of a quench-sensitive alloy such as 7075. Other alloys, such as 2024, are less sensitive to the effect of quench rate on mechanical properties but can be affected adversely by lowered resistance to corrosion as the quench rate is reduced. It has been found that in the case of 7XXX alloys [1] that quench rates greater than 100°C/sec offer no additional benefit to strengthening, but can drastically contribute to increased residual stresses and warpage.
The kinetics of precipitation occurring during quenching is dependent on the degree of solute supersaturation and the diffusion rate as a function of temperature. So, as an alloy is quenched, there is greater supersaturation (assuming no solute precipitates). But the diffusion rate increases as a function of temperature. The diffusion rate is greatest at elevated temperature. When either the supersaturation or the diffusion rate is low, the precipitation rate is low. At intermediate temperatures, the amount of supersaturation is relatively high, as is the diffusion rate. Therefore, the heterogeneous precipitation rate is the greatest at intermediate temperatures (Figure 2).
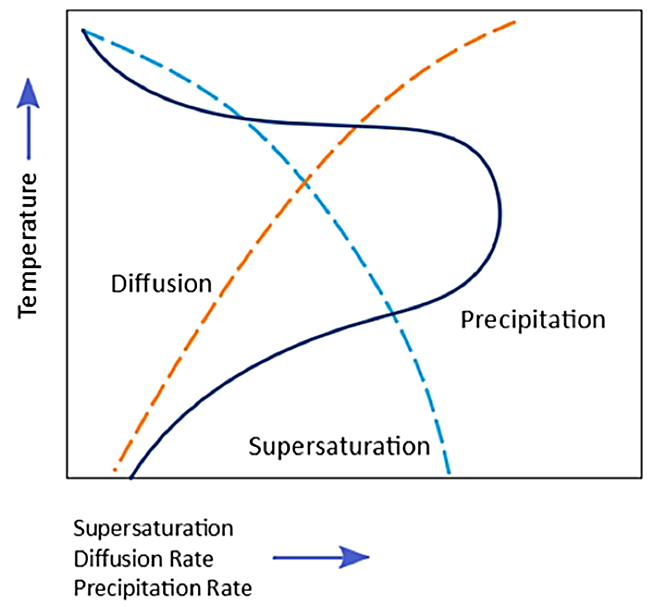
For most aluminum alloys, the critical temperature range is 400°C – 300°C. This means that quenching must occur rapidly through this range to prevent solute precipitation.
Quantifying quenching, and the cooling effect of quenchants, has been extensively studied [3] [4] [5] [6] [7]. The first systematic attempt to correlate properties to the quench rate in AlZnMgCu alloys was performed by Fink and Wiley [8] for thin (1.6 mm) sheet. An illustration of the effect of average cooling rate from the solution heat-treating temperature on tensile strength is shown in Figure 3. As can be seen, there appears to be a maximum average quenching rate of approximately 100°C/sec where little additional benefit from increased quenching rate is observed.
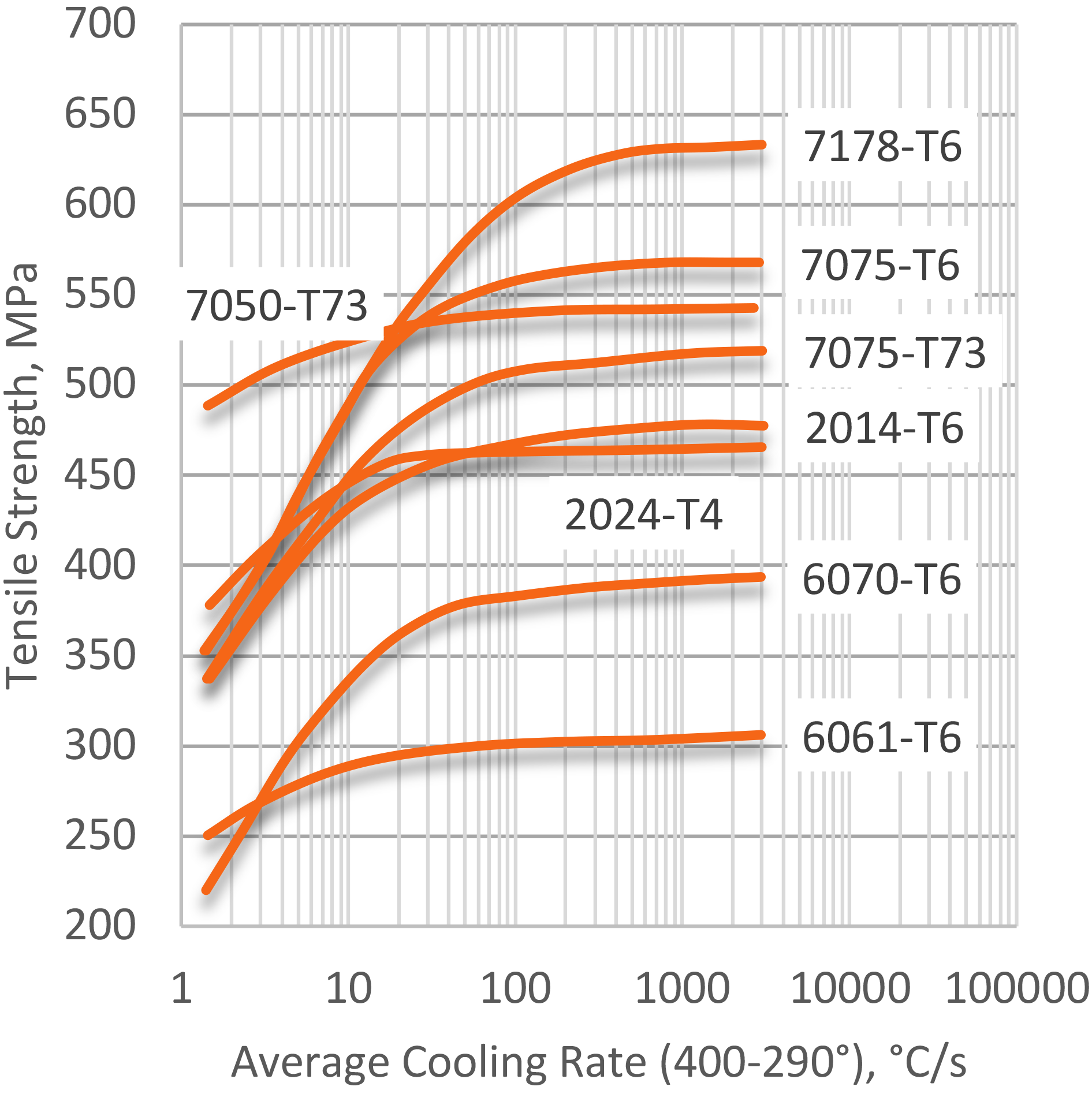
Average quench rates are useful in comparing experimental results from various quench methods. However, average quench rates only compare results in a “critical” temperature range, where precipitation is most likely to occur. This method is not entirely accurate, because significant precipitation can also occur outside the specified critical temperature range of average quench rates. Moreover, for high-strength alloys, toughness and corrosion resistance may be impaired without significant loss of tensile strength.
For most specifications used for aluminum heat treating, the delay time is measured from the time the furnace door is first opened, or the first portion of the load emerges from the salt bath, or the heating zone of a continuous, to the complete immersion of the load in the quenchant. The minimum thickness used to determine the allowable quench delay is the minimum dimension of the thinnest section of the load.
The specification of a maximum quench delay prevents the solution heat-treated load from cooling excessively during transfer to the quench tank. It ensures that the maximum properties are achieved. Exceeding the maximum allowable quench delay can result in a “slack quench,” and excessive precipitation of the solute on grain boundaries or other sites, preventing participation in subsequent aging.
Conclusions
In this short article, we discussed the basic metallurgy behind quenching aluminum. It is necessary to maintain the supersaturated solid solution down to room temperature. This is achieved by rapid quenching. In the next article, I will discuss quenchants for aluminum.
As always, should you have any questions or comments regarding this article, or have any suggestions for further articles, please contact the editor or the author.
References
- D. S. MacKenzie, Quench Rate and Aging Effects in Al-Zn-Mg-Cu Aluminum Alloys, Rolla, MO: University of Missouri – Rolla, 2000.
- J. E. Hatch, Aluminum: Properties and Physical Metallurgy, Metals Park, OH: American Society for Metals, 1984.
- M. A. Grossman, Metals Progress, vol. 4, p. 373, 1938.
- H. Scott, “Quenching Mediums,” in Metals Handbook, Cleveland, ASM, 1948, p. 615.
- F. Wever, Archiv fur Eisenhuttenwesen, vol. 5, p. 367, 1936.
- L. Dakins, Union Carbide.
- B. Liscic, H. M. Tensi, L. C. Canale and G. E. Totten, Eds., Quenching Theory and Technology, Boca Raton, FL: CRC Press, 2010.
- W. L. Fink and L. A. Wiley, “Quenching of 75S Aluminum Alloy,” Trans. AIME, vol. 175, p. 414, 1948.