This article is a follow-up to the three articles on “Mechanical Properties of Carburized Steel” in the March [1], April [2], and May [3] 2023, issues of Thermal Processing. In the March article, it was shown the carburized case by itself is not as strong as predicted by the hardness, and that it fractures with little or no plasticity. This is a result of quench embrittlement [4] due to the high hardness and high carbon content. Different methods to reduce quench embrittlement were also discussed.
In the April article, the development of two different carburized (case-core composite) 3-point bend test bars were discussed. The behavior was significantly different depending on whether a radius or stress concentration was present. The bending ultimate and yield (Johnson Elastic Limit or JEL determined by 50 percent change in slope) strength of the smooth bar increased with increasing core hardness. The bending ultimate and yield strength of the shouldered test bar with a radius increased with increasing core hardness but leveled off at 30-35 HRC, then the ultimate decreased to meet the yield strength at 40 HRC where it remained with further increasing core hardness.
In the May issue, the development of a carburized U-Notch test bar, which more closely represented an actual axle gear, was reviewed. The data once again showed the bending ultimate and yield strength increased with increasing core hardness and leveled off at 30-35 HRC, then the ultimate decreased to meet the yield strength at a core hardness of 40 HRC unless a higher alloy steel or a lower-case depth was used. The data also showed the bending strength without the carburized case was greater than with the case.
In this article, through-hardened core mechanical properties without the carburized case will be discussed, as well as the effect of alloy content, carburized case depth, and carbon content on strength.
Bending properties of core and transition zone
Figure 1 shows the bending ultimate and yield strength for a series of U-Notch test bars that were neutral hardened, oil quenched, tempered, and tested under 3-point bending [5].

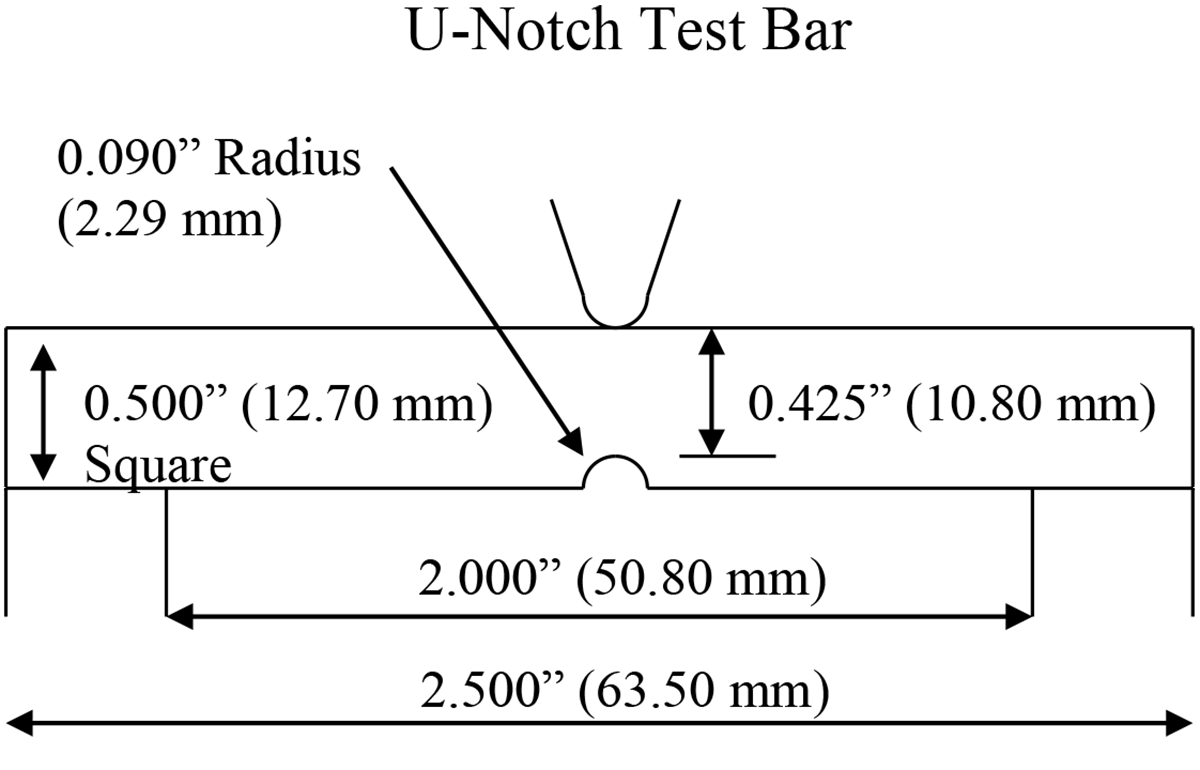
The dimensions of the U-Notch bar are shown in Figure 2. The steel grades used were 8615, 8620, 8630, 8640, 4320, and 9310. The bars were tested in the as-quenched (AQ) condition as well as with a 350°F, 600°F, 850°F, and 1,100°F temper. These samples were intended to represent the properties of the core and transition between the case and core.
The 8615 bending ultimate strength was 10,000 pounds for the AQ condition then increased slightly with the 350°F temper even though the hardness decreased slightly. This is likely the result of a minor degree of quench embrittlement. Figures 3 and 4 show the hardness of all samples at the surface and in the center of the core at the U-Notch respectively.

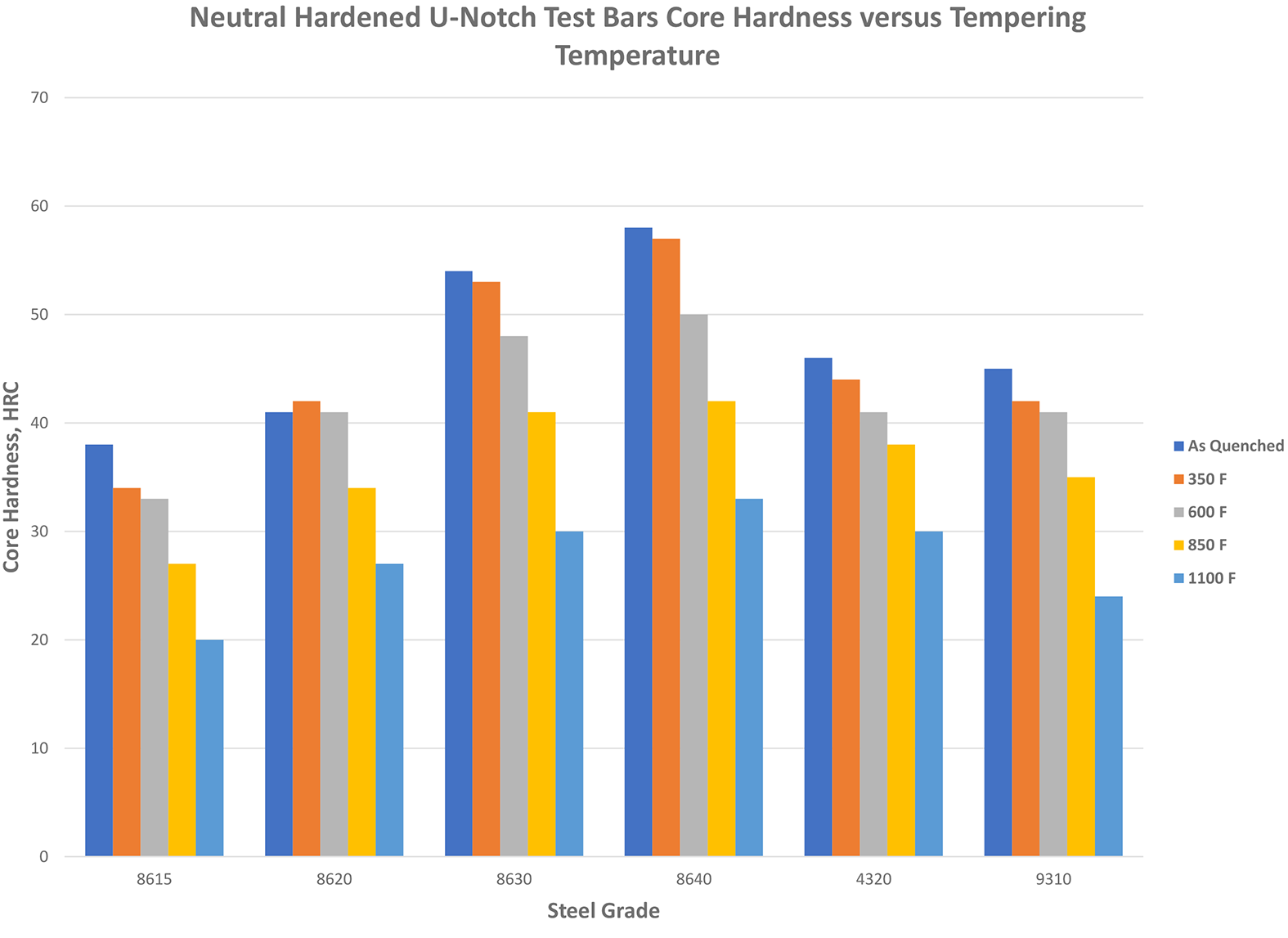
All samples were neutral hardened at the same time in the same batch. The furnace carbon level was set at 0.3%. As a result, the lower carbon steels had a slight case depth of about 0.005 inches with a surface hardness just more than 50 HRC. This low carbon case appears to have had no detrimental effect on bending strength as has been normal with a typical high carbon case. The bending ultimate strength with a 350°F temper was 10,400 pounds compared to 9,000 pounds in the May article. The May sample core hardness was 31 HRC and was copper plated rather than neutral hardened, so no case was present. The core hardness of the current sample was 34 HRC. The 8615 bending ultimate strength then further decreased for the 600°F, 850°F, and 1,100°F tempering temperatures as the hardness continued to decrease.
Steel normally has a very good relationship between hardness and ultimate tensile strength. As hardness increases, the ultimate strength also increases. Quench embrittlement can negatively affect this relationship especially at high hardness and elevated steel carbon levels. Essentially, the plastic portion of the stress strain curve is reduced or eliminated causing the steel to fail in a brittle manner. Typically, embrittlement can be eliminated by tempering at 600°F, but this also reduces the hardness, strength, compressive residual stress, and fatigue life.
Returning to Figure 1, 8620 steel with a higher hardness compared to 8615 would be expected to have a higher strength. However, in the AQ condition, the ultimate strength is lower than the 8615, and the deflection is also lower. This is a result of quench embrittlement. Figure 5 shows the deflection for all samples. The 8620 ultimate and yield strength is greater than the 8615 for all the remaining tempered samples. The strength is maximum at the 600°F temper and then decreases with increasing tempering temperature as the hardness also decreases.
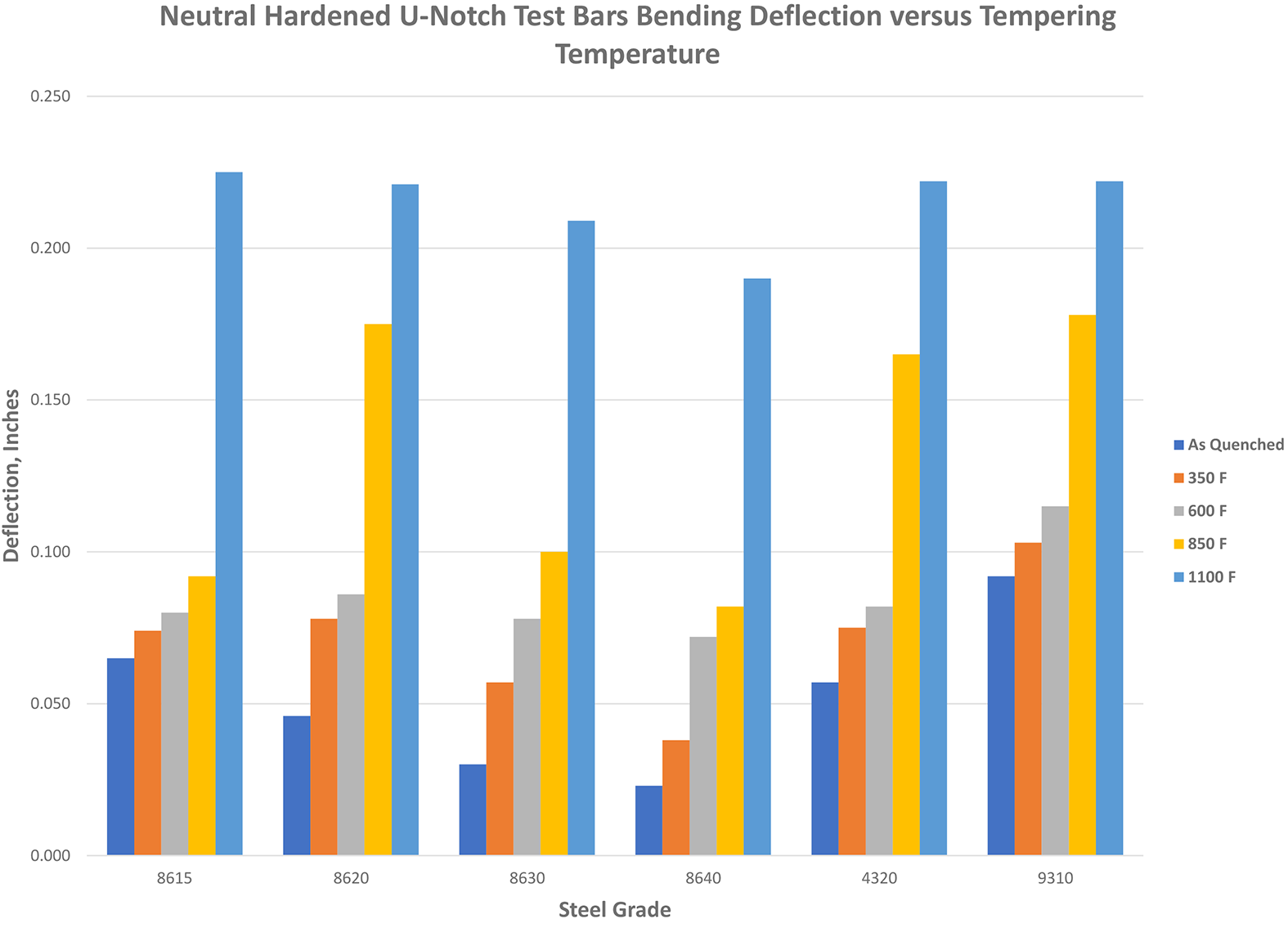
The 8630 steel continues the same trend as the 8620 except the embrittlement in the AQ condition is more significant, and the spread between ultimate and yield strength has almost completely disappeared. A small reduction in strength is also present for the 350°F condition. The strength of the remaining tempered conditions is greater than the 8620 steel due to the higher hardness.
The 8640 steel continues the same trend as 8630, but now the AQ and 350°F temper conditions have become severely embrittled. The negative effect of increasing steel carbon level is evident with this sample configuration in bending. From this, it is easy to see why the carburized case at 0.80-0.95% carbon would suffer from quench embrittlement.
The next two steels in Figure 1 are 4320 and 9310. The first is a medium nickel steel, and the second is a high nickel steel typically used in the aircraft industry and for demanding applications elsewhere. The 4320 steel has higher strength and deflection compared to the 8620 only in the AQ condition. The 9310 steel has higher strength compared to the 4320 only in the AQ condition and higher deflection in the as-quenched 350°F and 600°F temper conditions. The ultimate core bending strength of 8620, 4320, and 9310 with a typical 350°F temper is the same. The only differences are the deflection increases with increasing alloy content for the AQ 350°F and 600°F temper conditions, and the AQ embrittlement is reduced.
Bending properties vs. alloy content
In the May article, there was a significant increase in bending strength and low cycle bending fatigue life for case carburized 9310 versus 8615 or 8620 steel. The 4320 steel also had the capability to provide increased performance depending on the core hardness and case depth. The U-Notch through-hardened core data does not seem to predict any increase in strength for 9310 and 4320 over 8615 or 8620, other than in the AQ condition.
Tensile properties of core vs. alloy content
Figures 6 is core tensile and yield strength data vs. hardness from the AISI database [6] referenced in the March article. Tensile strength vs. hardness for four steels with increasing nickel content is shown. The no-nickel steel was 20MnCr5; the low-nickel steel was 8620; the medium-nickel steel was 4320, and the high-nickel steel was 9310. Three different hardness levels of each steel grade were tested. The hardness was controlled by the rough sample diameter that was vacuum pseudo carburized, oil quenched, and tempered at 350⁰ F. Test bars were then machined from the rough samples. The ultimate tensile strength depends only on hardness, and the alloy content makes no difference. The high nickel steel doesn’t provide any higher strength than the no nickel steel.
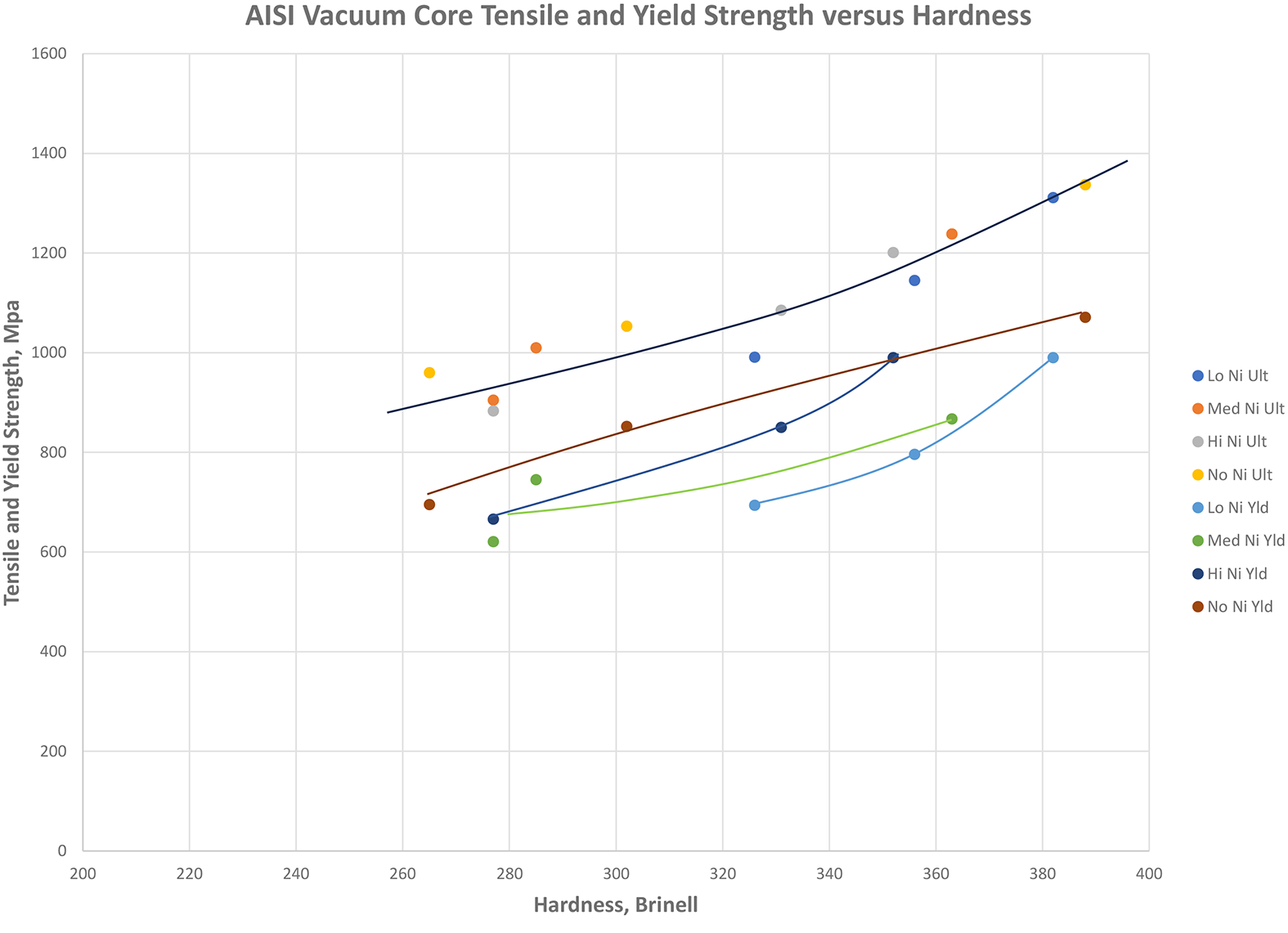
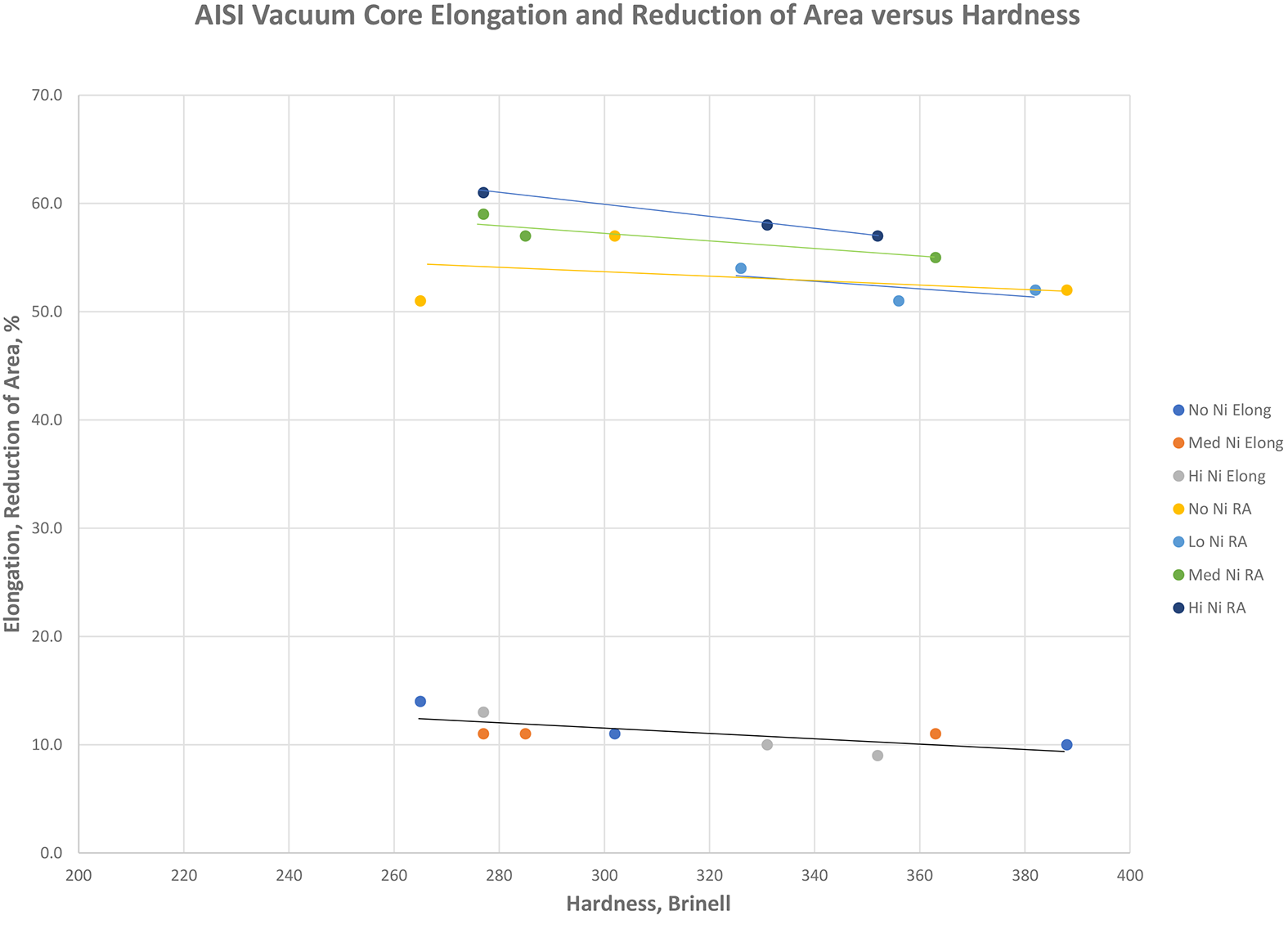
Figure 7 is elongation and RA data vs. hardness for the different steels. The elongation exhibits dependence on hardness but not on alloy content. There, the low nickel elongation data was not included due to a measurement error. However, the RA does indicate some dependence on the alloy content. The high-nickel steel has the highest RA followed by the medium nickel steel, and the low-nickel and no-nickel steels are the lowest and are both about the same. Like the U-Notch bars tested in bending, the tensile data shows the only difference between grades is increasing RA with increasing nickel content.
Tensile properties of case vs. alloy content
The AISI Bar Fatigue data referenced in the March article also included tensile strength for through-carburized test bars made from different steel alloys. Figure 8 shows tensile strength data for no-nickel 20MnCr5, low-nickel 8620 and 8695, medium-nickel 4620 and 4320, and high-nickel 9310 steel grades. There is too much scatter or variation in the old data to make any conclusions. However, more recent data does show a correlation. These samples were all vacuum-through carburized on the same 36-hour cycle and tempered at 350°F. The samples were machined and polished prior to vacuum carburizing, and no hard finishing or cleaning was done prior to testing. From a percent standpoint, there is a reasonable increase in strength from the no-nickel steel to the high-nickel steel. However, these values are all very low compared to the strength of the core. This, too, does not seem to explain the reason for the increase in case carburized bending strength as the alloy content increases.

Bending properties with and without case, effect of alloy and case depth
Figure 9 shows the difference in bending ultimate and yield strength for carburized U-Notch Test Bars with a carburized case vs. without from this article and the May article. The steel grades are 8615, 8620, 4320, and 9310. The core hardness is shown above the bars. It is evident the “without case” data on the right-hand side is significantly higher in strength compared to the “with case” data on the left-hand side.
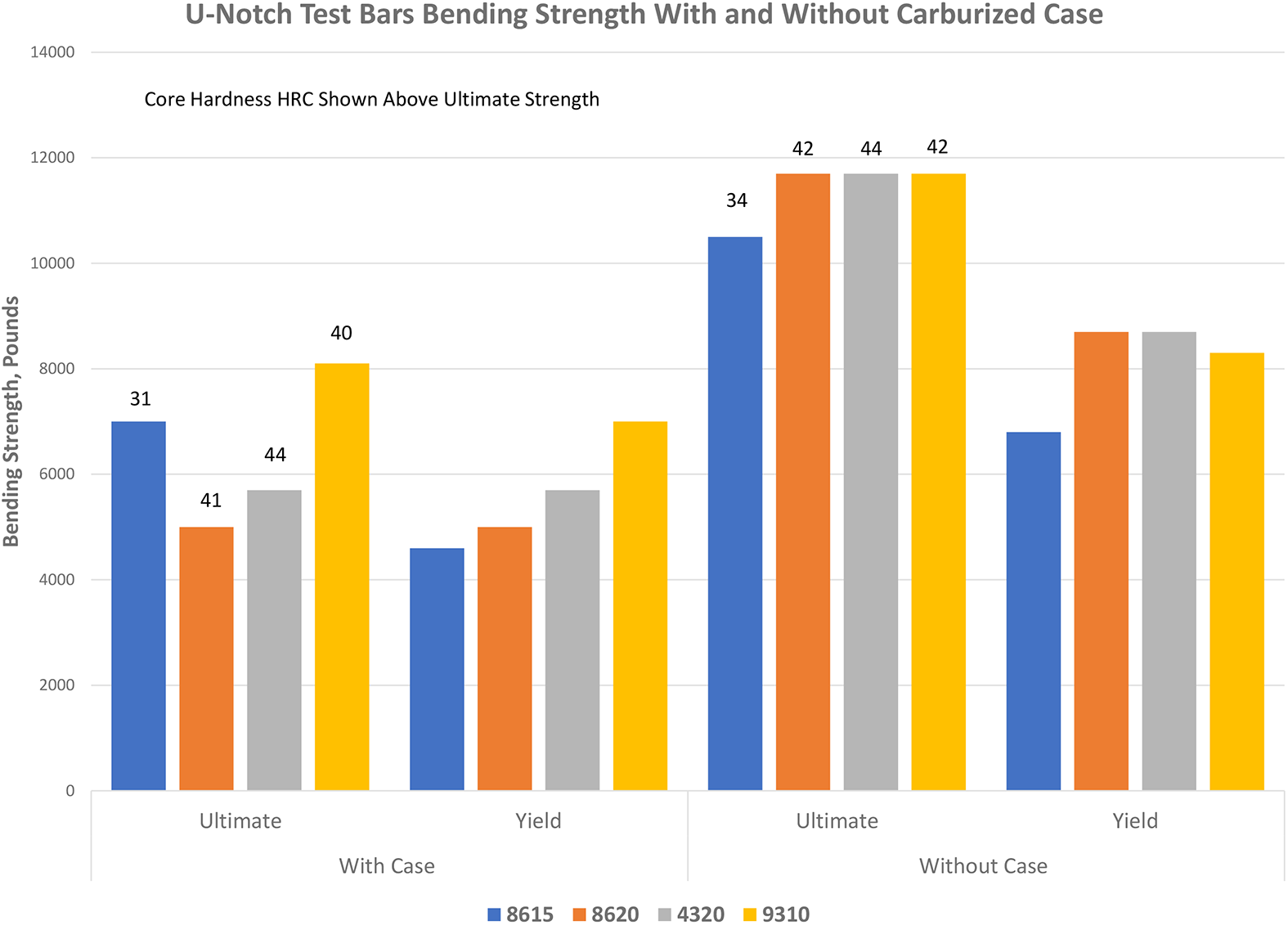
The carburized case is detrimental toward bending strength in the presence of a radius at the surface. The case is not as detrimental with 8615 with a core hardness in the 31-34 HRC range compared to the 8620 with a core hardness in the 41-42 HRC range. The 4320 was able to provide a little higher bending strength than the 8620, and the 9310 was even better. The strength of the case carburized U-Notch bars appears to be more than the sum of its parts. The properties of just the case or just the core do not predict what happens with the case core composite.
Figure 10 provides additional data to demonstrate the effect of the carburized case on bending ultimate strength from this article and the May article. The core hardness values are shown above the bars. The four blue bars on the left side of the chart show through-hardened 8600 steels with increasing carbon content and no case. The steel grades are 8615, 8620, 8630, and 8640. As the steel carbon content increases from 0.15% to 0.20% to 0.30%, the hardness and bending strength increased from 10,500 pounds to 11,700 pounds to 12,800 pounds. However, as the hardness further increased to 56 HRC at 0.40% carbon, the bending strength decreases to 8,700 pounds. This is a result of quench embrittlement, which becomes more severe at higher carbon levels. The orange bars on the left side of the chart show corresponding case-carburized data for 8615, 8620, and 8625 steels with an approximate effective case depth of 0.035 inches. The 8615 provided the highest bending strength at 7,000 pounds with a core hardness of 31 HRC. As the core hardness increased to 40 HRC and above for 8620 and 8625, the bending strength dropped to 5,000 pounds and remained constant. This relationship was presented in the May 2023 article.

On the right side of Figure 10, data is shown for 4320 and 9310 steels. The blue bars are through-hardened with no case depth; the orange bars are standard case carburized with an approximate effective case of 0.035 inches. The gray bars are light-case carburized with an approximate effective case of 0.016 inches. The through-hardened bars with no case depth are considerably stronger than the standard case carburized bars for both 4320 and 9310. The standard case-carburized samples have the lowest bending strength, and the light case-carburized samples provide a significant increase in strength. The case-carburized samples for the 9310 provided a significant increase in strength compared to the 4320 as well as the 8615, 8620, and 8625.
Effect of case carbon on bending
Data was published in 2009 showing the effect of case carbon content on the bending strength, bending and contact fatigue life, and impact life of carburized gears and U-Notch test bars [7]. Figure 11 shows the bending strength vs. case-carbon content for 8620, 4320, and 9310 U-Notch test bars.
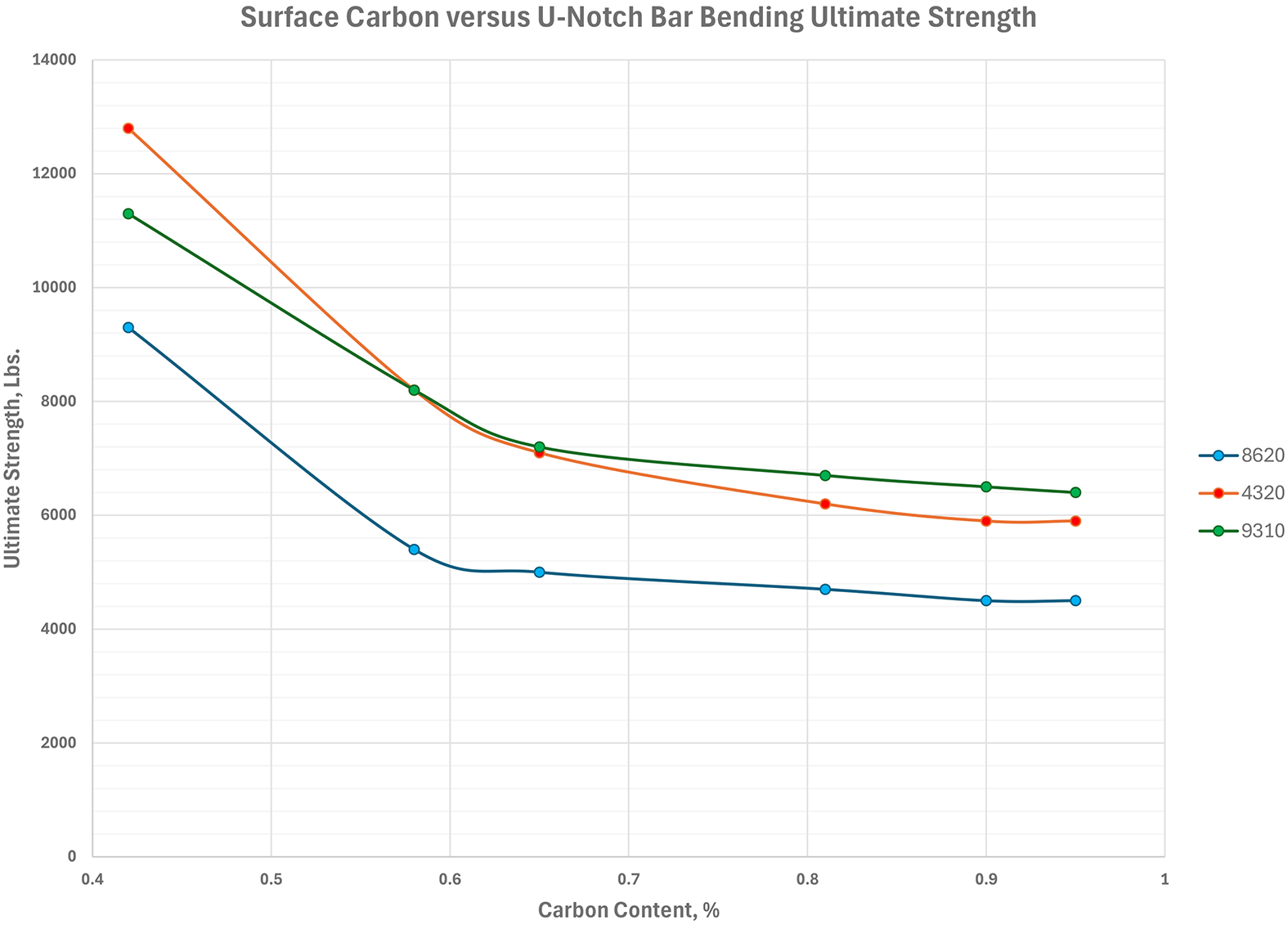
All test bars for each carbon level were carburized at the same time in the same batch with a target of 0.035 inches effective case depth for the 8620 samples. The bending strength at the typical 0.80-0.95% carbon level is relatively constant. The 8620 steel is at 4,500 pounds while 4320 is at 6,000 pounds and 9310 is about 6,500 pounds. As the carbon level decreased to 0.65% and 0.58%, the bending strength gradually increased for each steel. Below 0.60% carbon, there is a more significant increase in bending strength. At 0.42% carbon, the bending strength is double that at 0.80-0.95% carbon. The 8620 steel is now at 9,300 pounds while 9310 and 4320 traded places and are at 11,250 pounds and 13,000 pounds, respectively. For comparison, the neutral-hardened 8615 sample in Figure 1 with a shallow 0.30% carbon case had a bending strength of 11,400 pounds. It is also interesting to note the through-hardened 8640 sample in Figure 1 is nearly the same strength as the 2009 case carburized 8620 sample with a surface carbon of 0.42%. Both samples had the same surface carbon, but the core hardness was 57 HRC vs. 42 HRC respectively. According to the shouldered test bars in the April article and the U-Notch bars in the May article, once the core hardness exceeds 40 HRC the bending strength remains low and constant with no ductility.
Summary
The strength of the carburized case likely varies considerably from the surface into the core. The high hardness high carbon outer portion appears to be brittle and relatively weak. In terms of tensile strength, it is about 1,100 MPa or 160 Ksi. As the carbon content decreases toward the core and the hardness is still relatively high, the strength likely increases by a significant factor. The highest strength is likely around the effective case depth at 0.30% carbon with a hardness just above 50 HRC. This corresponds to a tensile strength around 1,900 MPa or 275 Ksi. The strength then decreases toward the core. At a core hardness of 33 HRC, the tensile strength would be about 1,035 Mpa or 150 Ksi. which is close to the outer-case properties. All of this happens in a relatively short distance. In bending, the carburized case typically cracks at or below the yield strength, and the remainder of the cross section continues to support the increasing load up to the ultimate. This is likely a function of the fracture toughness of what is remaining at that point. At the completion of successful vehicle durability testing, it is not uncommon to find cracks in the carburized case if abuse events have been included. Surprisingly, cracking of the case doesn’t mean the vehicle can’t live a long useful life.
It is important to remember what has been discussed here relates to carburized steel bending loads or stresses. To function properly, many carburized components must also account for contact or surface loads. What is good for bending may be the opposite of that which is good for contact. This may require a compromise to satisfy both requirements.
References
- G. Fett, “Carburized Steel Mechanical Properties Case Tensile Strength,” Thermal Processing, March 2023, Pages 33-35.
- G. Fett, “Carburized Steel Mechanical Properties Bending Ultimate and Impact Strength, Test Bars Vs. Actual Parts,” Thermal Processing, April 2023, Pages 45-47.
- G. Fett, “Carburized Steel Mechanical Properties Bending Ultimate and Impact Strength,” Thermal Processing, May 2023, Pages 39-41.
- J. Wong, D. Matlock, G. Krauss, “Effects of Induction Tempering on Microstructure, Properties and Fracture of Hardened Carbon Steels,” Proceedings of 43rd Mechanical and Steel Processing Conference, ISS, October 2001.
- Unpublished work done by G. Fett, courtesy of Dana Corporation, circa 2007.
- https://fde.uwaterloo.ca/Fde/Materials/SMDIdbase/Steel/references.html.
- G. Fett, “The Effect of Carbon Content on the Performance of Carburized Steels,” Proceedings of the 25th ASM Heat Treat Conference, September 2009.