Film quality, throughput, yield, etch uniformity, and sidewall control all depend on the ability to ignite, maintain, and control a stable plasma. Precise, nimble power delivery is crucial. Extremely tight accuracy is required for the stringent multi-step manufacturing processes that deposit or etch material to produce near-flawless nanostructured films on a fragile glass substrate or transform a silicon wafer into thousands of microcircuits and billions of transistors — potentially worth hundreds of thousands of dollars.
The application-specific requirements of etch and deposition processes demand a wide range of products and capabilities that can operate with exceptional reliability and stability, addressing specific challenges of fabricating nano- and micro-scale electronics. Plasma characteristics are dynamic; power supplies must continuously respond to instant changes to support a stable plasma, while fulfilling the performance requirements of the specific process recipe. These recipes could include hundreds of steps, each requiring different plasma conditions in quick succession; as such, the capability to shape plasma characteristics quickly and precisely becomes more and more critical. The power supply’s inherent topology, algorithms, controls, and sensor capabilities determine its ability to perform within these extremely tight parameters.
Plasma Ignition and Maintenance
The initial voltage required to ignite a plasma depends on many factors, including chamber geometry, gas chemistry, pressure, temperature, and other conditions. For example, the Paschen curve shown in Figure 1 illustrates the dependence of ignition voltage on pressure and distance between electrodes for DC breakdown.

To reliably and repeatably achieve plasma ignition, the power supply should be able to deliver a precise voltage “spike” within these parameters. This accelerates the electrons within the plasma chamber, providing enough kinetic energy to collide with and ionize the process gas atoms or molecules. An initial number of free electrons collide with additional atoms to release further electrons, creating an avalanche effect.
Once the correct conditions are achieved, ignition occurs in a matter of microseconds to milliseconds, causing drastic changes in the process environment, including impedance. Because the voltage required to sustain a plasma is less than that required to ignite it, the power supply must react almost instantaneously to decrease voltage to processing levels. Without a high degree of speed and responsiveness, the plasma can extinguish or become unstable. Plasma impedance is highly dynamic, and significant changes can occur. For example, it may change during ignition when pressure variations (gas dissociation) occur as the system stabilizes, when system drift occurs as electrodes are heated over time, and under other conditions.
Today’s advanced power supplies are able to swiftly and continuously react to conditions within the process environment to support plasma stability. The graphic in Figure 2 illustrates the closed-loop relationship in an RF-powered process between plasma characteristics and power-delivery precision and responsiveness, which applies both to the pre- and post-ignition phases; dP represents incremental changes in delivered power that affect plasma properties, such as impedance, dZ. In turn, changes to the plasma environment necessitate changes to power delivery.

The plasma-power generator must both optimize power delivery considering known factors and respond almost instantaneously to a dramatic shift in conditions within the chamber once the plasma ignites. For example, empirically determined match-network presets “predict” the impedance of the plasma before it ignites.
Speed and agility are key not only to immediately post-ignition, but also throughout processing — with different requirements depending on the process type and during any type of transition within an active plasma process.
Plasma-Enhanced Chemical Vapor Deposition (PECVD)
In multi-step PECVD processes for semiconductor manufacturing where every nanometer matters, the RF power supply must react in microsecond time scales to changing process environments as described above. In a PECVD process, chemical reactions inherent in this deposition technique cause plasma impedance fluctuations as new process gases are introduced and residual contaminants are pumped out. As chip architectures become smaller and more complex, the production process steps become shorter and more frequent and the power supply, too, must be more agile to maintain the specific plasma characteristics required for a given process step and application.
Etch
As device structures shrink, etch dimension precision, profile/uniformity control, and end-point detection become increasingly critical. Similar to PECVD processes, the ability of an RF power system’s impedance matching algorithms to quickly and continuously tune power delivery to match the dynamically changing complex impedance of the plasma is key in etch processes. Besides plasma changes, etch surfaces also undergo dynamic electrical and chemical changes as they are depleted of material, which can affect power-delivery stability. As in any RF-powered process, but especially with the unique factors within etch applications, fast tuning algorithms are key to responding quickly and precisely adapting power delivery to maintain process stability and ensure maximum power delivery into the process environment.
Etching of contact holes or high-aspect-ratio trenches, especially on insulating materials, can be accompanied by undesired charging effects that are responsible for micro-trenching, notching or twisting of structures, which in turn negatively affect component performance. Charge accumulation generates electric fields that can distort incoming ion trajectories and can lead to breakdown of the dielectric layer. Power-modulated/pulsed plasma with a variety of pulsing combinations (source, bias, synchronous etc.) has been used to control radical/ion fluxes and energies to optimize conditions to resolve above challenges. (Figure 3)
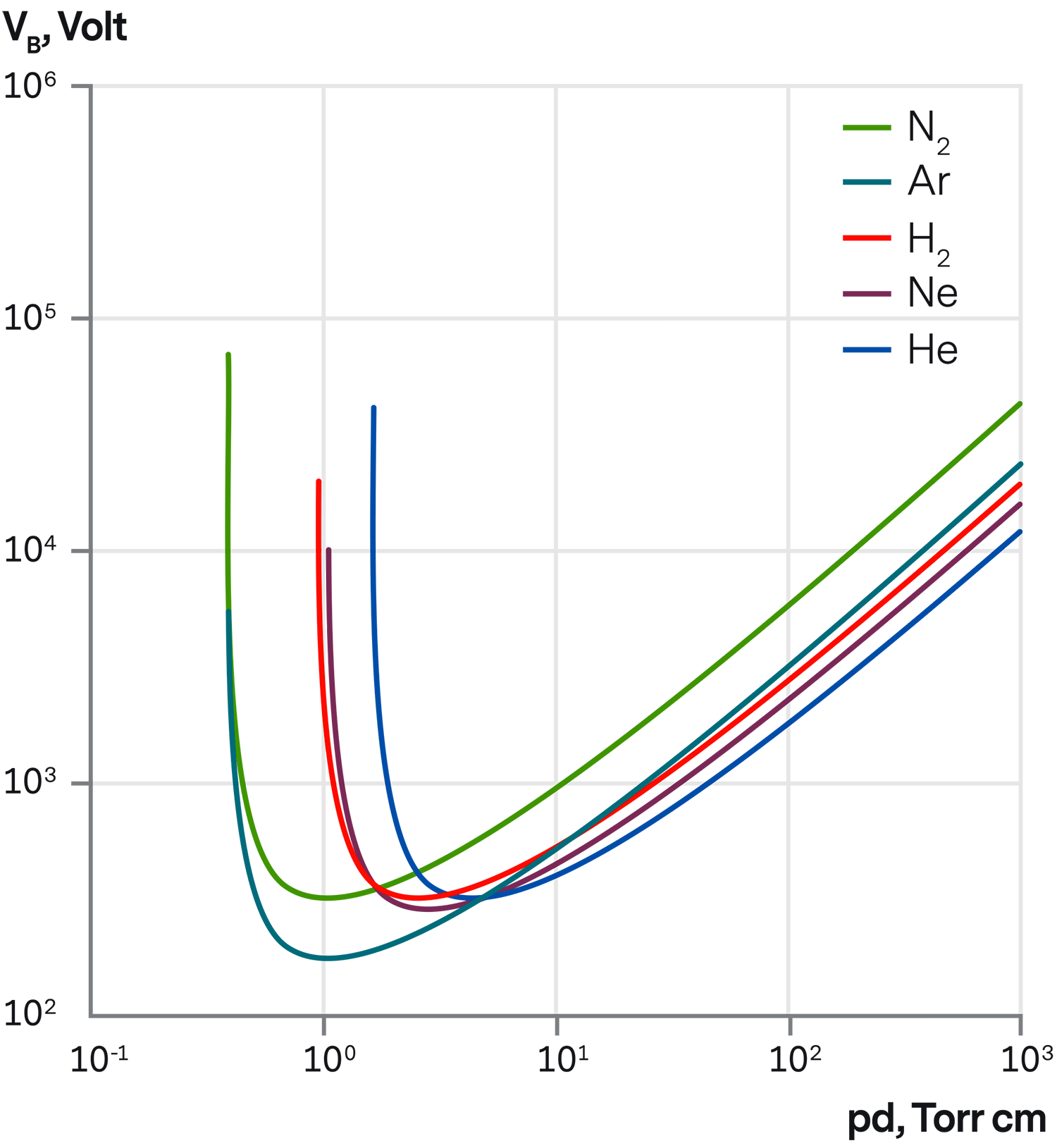
Pulsing also allows access to energy regimes that typically are not available with continuous wavelength (CW) operation, providing more precise control of certain etch and species (byproducts) and thus better uniformity. It enables control of the ratio of high- and low-energy ions, as well as manipulation of ion-energy distribution function (IEDF) outside of allowed limits for CW.
Physical Vapor Deposition (PVD)
Demanding applications, such as the following, require the power supply react to process instabilities in microsecond time scales:
• Deposition of high-quality TCO coatings, such as ITO, IGZO, AZO, etc., from multicomponent targets on flexible/heat sensitive substrates.
• High-hardness coatings, such as metal nitrides and carbides on work pieces.
• Large-area coatings of uniform and defect-free insulating materials such as SiO2 and TiO2.
• Decorative coatings with high-quality color and surface finish.
To maintain plasma stability and deposition quality, the power supply must be able to regulate multiple parameters, including power, voltage, and current, while adjusting power delivery within a fraction of a millisecond.
Arc Management
Arcing is a common form of plasma instability in deposition processes that happens when built-up charges around oxides or inclusions on a target surface suddenly discharge. If not managed quickly and effectively, this can have potentially devastating effects, including substrate (cracks, defects at edges), target (nodules formation), and equipment damage. When an electrical discharge occurs, the arc can eject particulates from the target, which can cause film damage such as macroparticles, pinholes, inclusions, and other structural surface defects. Arcs also can drain energy from the total amount supplied to plasma system.
Several aspects of a process affect arc rates and arc energy, including delivered power level, target material, process gas, chamber geometry, and other factors. Though various aspects of the plasma system can lead to arcing, the power supply is key to minimizing process disturbances associated with damage, with different functionality depending on process-power type.
Arc Detection and Handling
Monitoring of both current and voltage enables today’s plasma power generators to detect an arc quickly and accurately. A robust modifiable arc management procedure should be available to users so that they can manage the arc treatment according to their specific requirements. Especially in reactive sputtering of dielectric material, pulsing and proper arc management have led to strong reduction in arc-related damage on coatings.
In a DC-powered process, during an arc event, changes in plasma impedance cause the output voltage to quickly approach zero,
while the output current significantly increases. An increase in voltage and/or current beyond a defined limit or set of threshold criteria initiates an immediate power-supply response. In as little as 1 µsec, the power supply first reverses voltage to swiftly dissipate the current driving the arc, dropping to zero A in a 3-to-4 µsec timeframe.
This voltage reversal is followed by a shutdown phase during which power delivery ceases. The duration of the shutdown is brief (tens of micro-seconds) and precisely timed to completely extinguish the arc without causing process instabilities that could affect throughput and yield. If this response is successful, the event is called a micro-arc. However, if the arc persists, it is termed a hard arc, and the power supply shuts off for a progressively longer duration until the plasma stabilizes. The ability to adapt to specific process conditions helps minimize power-delivery shutoff time to maintain delivered power and throughput. In addition, power supplies that are able to slowly ramp energy back up after an arc-response shutdown reduce the likelihood that the arc will be re-ignited by applying too much energy too soon.
RF processes are typically less susceptible to arcing than DC due to the nature of the technology; however, certain arc management algorithms exist for particularly arc-prone processes.
Arc Energy
Current market demands for higher throughput are raising the pressure to increase process power levels. However, as process power levels increase, arc energy (expressed as mJ per kW) also increases because the energy available to an arc is determined by the energy stored in the output of the power supply plus that stored in the cabling. Providing less power to the arc reduces the likelihood it will cause damage before the power supply’s response can extinguish it. Therefore, power supplies designed to minimize stored energy generally are associated with less arc damage within the process.
Arc Prevention
Bipolar DC technology, typically used in PVD processes for industrial coatings, can neutralize and/or prevent charge buildup by dissipating it on the reverse pulse. This regularly shuts plasma off by reversing voltage (for example, every 50 kHz) to clear “hot spots” on the target. While pulsing benefits processes by using time dependencies to enhance effects (e.g., broad energy distribution of electrons at the start of the pulse), the periodic reversal of voltage of an electrode helps clear charge buildup and thereby arcing.
Though RF-powered processes typically are less prone to arcing, pulsing also can prevent charge buildups that lead to damaging electrical discharges in these applications.
Process Visibility
Access to high-resolution data and analysis opens a window into actual conditions within the plasma system in real time, providing insight into power-supply and process performance. DC and RF power supplies that come with embedded or optional add-on solutions for data acquisition, as well as available data analysis consultation, enable process operators maintain a more stable, repeatable plasma and process. Browser-based dashboards, high-data resolution, and sophisticated algorithms increase the speed and accuracy of process fingerprinting and root-cause analysis of process deviations, helping users pinpoint ways to resolve existing issues and visualize previously unidentified optimization opportunities.
Such a platform can expand process, machine, and quality data along five key parameters:
- Visibility.
- Resolution.
- Accuracy.
- Aggregation.
- Analysis.
With the unique knowledge provided by this solution, plasma process operators can pinpoint ways to stabilize the plasma and process and visualize previously unidentified optimization opportunities over the long term. (Figure 4)

Conclusion
The ability to establish and maintain a stable plasma hinges on choosing the right power supply for your application and process — not only the right type of power, but a unit that can provide features and functionality for specific film quality, etch quality, throughput, and yield requirements. Power-delivery agility and plasma control are increasingly critical, especially in semiconductor applications as device architectures shrink, processes transitions become faster, and recipes become more complex. With high-quality topologies, fast algorithms, sophisticated controls, and extensive sensor capabilities, the most advanced power supplies keep pace with today’s quickly evolving technologies.
About the author
Dhaval Dhayatkar is senior director of marketing at Plasma Power.