
Ceramic refractory materials are useful for the following functions:
1. Serving as a thermal barrier between a hot medium and the wall of a containing vessel.
2. Withstanding physical stresses and preventing erosion of vessel walls due to the hot medium.
3. Protecting against corrosion.
4. Providing thermal insulation.
Refractories have multiple useful applications. In the metallurgy and ceramic industries, refractories are used for lining furnaces, kilns, reactors, and other vessels which hold and transport hot mediums such as metal and slag. Refractories have other high temperature applications such as fired heaters, hydrogen reformers, ammonia primary and secondary reformers, cracking furnaces, utility boilers, catalytic cracking units, air heaters, and sulfur furnaces.
This article will review Insulating Firebricks (IFB), which are a type of thermal insulation bricks which are shaped refractory products with an application temperature of greater than around 1,000°C and a total porosity greater than 45 percent. The IFB is a relatively soft brick made of refractory ceramic material that can withstand high temperatures with low thermal conductivity. IFBs are generally light in weight and can be easily cut by handheld hack saw or any other hand tool such as a chisel or drill. They are ideal for creating custom shapes, curves, and cavities. However, due to the large apparent porosity, loose structure, low strength, as well as poor resistance to various types of erosion and wear resistance, They can only be used more in the heat insulation layer of kilns and other high-temperature thermal equipment. Generally, IFBs are not suitable for work lining and heavy load-bearing structures or environments with aggressive erosion and wear.
The majority of commercially available IFBs are alumino-silicate refractory bricks, silicate bricks, or lightweight corundum (alumina) bricks. The chemical composition has a direct influence on the classification of the refractory bricks. In general, the higher the alumina content and the firing temperature of the insulating firebrick, the higher its classification temperature.
The most common raw materials used for IFB include clay, kaolin, kyanite, mullite, (light) chamotte, sillimanite or andalusite. The thermal conductivity is influenced by the ingredients as well as the total porosity, pore shape, and pore size distribution. The porosity is produced by burnout materials and water. Common burnout materials include sawdust, straw, styrene bubbles, coke, or cellulose. In addition, foam or foaming agent can be added to increase porosity. Soap or saponins are used as foams, while metal or carbide powders are used as foaming agents in the casting processes. Lightweight bricks with high porosity can be produced this way.
Raw materials and burnout materials are optionally mixed dry and/or mixed with water. The amount of water depends on the shaping process. Different suppliers manufacture IFBs by different techniques including casting, slingering, extrusion, or dry pressing. These different techniques can result in a variety of properties and insulation qualities.
For the casting process, bricks are direct cast into large molds often made of plaster, with vibratory aids to assist flow. Water-binding raw materials extract or gel water from the slurry and support the setting of the green body. The gelling process can be accelerated by adding gypsum or cement to the mix. Due to the high water content, the drying of the green cast compacts can take a correspondingly long time. Casting is used for larger or more specialty shapes of lower to moderate volume.
The “slingering” process is a continuous process in which the masses are slingered in large molds or on a conveyor belt. The slinger process is a form of low-pressure extrusion of a wet clay mix containing high levels of burnout additives with the additional processing step that the semi-extruded material gets slung onto a continuous belt to generate additional porosity before drying and firing. Insulating firebricks of medium density can be produced this way.
The extrusion process forces a damp clay mixture containing burnout additives through an extrusion nozzle, where the extrudate is subsequently cut into bricks, dried, and fired.
Dry pressing is usually done uniaxially. The pressing method is suitable for the production of bricks with a higher density, so is more often used for high-density bricks.
The shaping process and the porosity agents cause typical porous structures of the insulating firebricks. This leads to a wide variety of thermal conductivities within the same class of product, which in turn leads to some variation in the ability of the different types of IFBs to control energy loss from the kiln. (Table 1)

In the IFBs, low density goes along with low strength. The cold compressive strength is specified as a characteristic value. The “hot” properties of the material must be good enough to support walls and arches at the desired application temperature. Hot bending strength or creep under load are characteristic values for the use at high temperatures.
Firing of the refractory bricks is often done in shuttle batch or tunnel continuous kilns. The firing temperature is equal to the specified classification temperature for each IFB mix. The IFB shapes do shrink during drying as well as during firing. This results in most bricks needing to be cut and/or ground to final size and shape after firing.
The different manufacturing methods for IFBs produce products with differing structure and chemistry, which in turn deliver different performance properties. The primary performance parameter for IFBs is the ability to insulate, which, in terms of measurable properties, is assessed by the thermal conductivity of the product. Density is sometimes used as a rule-of-thumb indicator of the insulating ability of an IFB, but this can be misleading.
IFBs are classified in the United States into different groups according to ASTM C155 guidelines (Table 2). The grouping results from the classification temperature and the density of the IFB. IFB of each group may not exceed a defined density and may not exceed a shrinkage of two percent after firing for 24 hours. The test temperature is 30°C below this classification temperature.
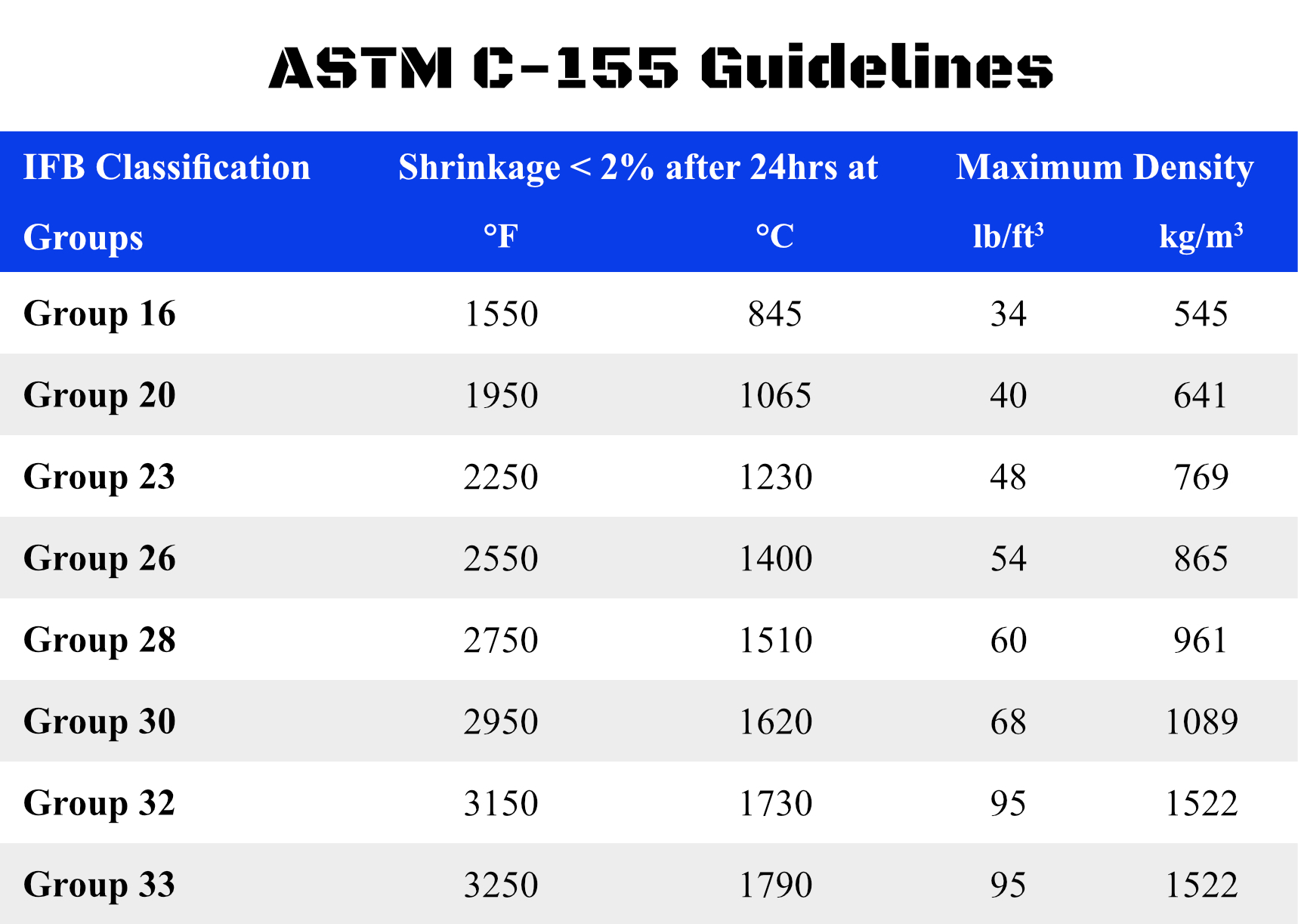
As discussed, the properties of the IFBs result from the chemical composition, the pore formers/density and the firing temperature. Higher alumina (Al2O3) content and higher firing temperature of the IFB result in a higher classification temperature. Take the group number and multiply it by 100 to get the rated classification temperature. Note that the upper maximum application temperature of the materials is usually about 100°C below the classification temperature.
Table 3 contains a list of typical properties for standard IFBs commonly available from Groups 23 through 32.
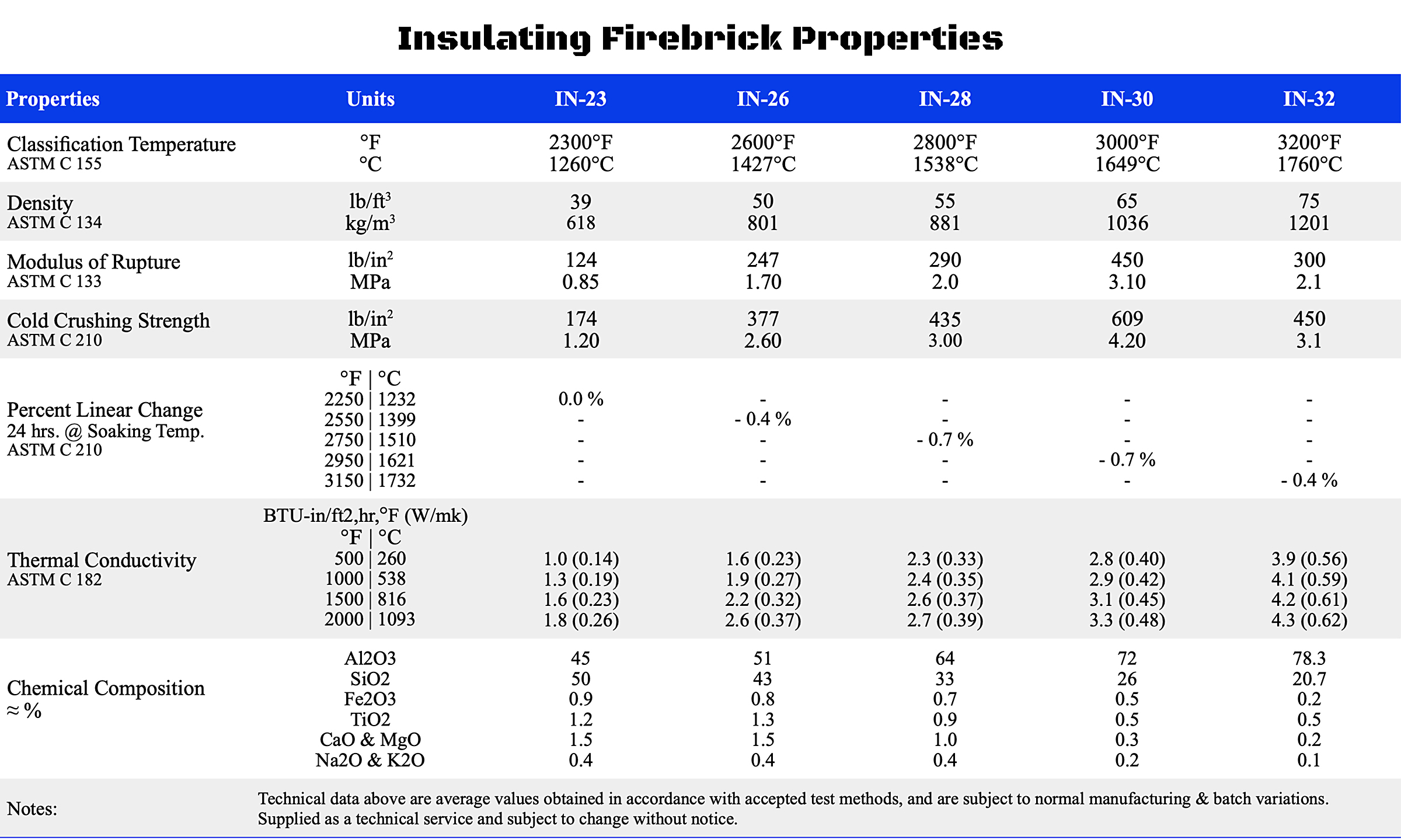
The thermal conductivity of the final product depends on the chemical composition, density, and pore structure. Insulating firebricks with low density and fine pore structure have a low thermal conductivity. The pore structure and density are largely determined by the manufacturing process. Fine burnout materials and a high water content in the process lead to a finer structure of the firebrick. This can be supported by using a foam/foaming agent. However, the density cannot be reduced endlessly. There must be sufficient structure in the green body to dry and burn it crack-free.
Table 4 shows typical thermal conductivities of different IFB groups.

IFBs are normally used in applications >1,000°C (1,832°F) because, at these temperatures, they provide the most cost-effective insulation available compared to alternative insulating refractories. The structural nature of the products also means that they offer better resistance to abrasion in high-temperature environments, coupled with chemical resistance (when the chemistry is tailored to cope with specific gases) compared to ceramic fiber insulation.
At application temperatures above 1,000°C, the most important heat-transfer mechanism becomes radiation rather than conduction and convection, which are the more significant heat-transfer mechanisms at lower temperatures. The large pore sizes in the cement IFB are inefficient at retarding energy transfer at the infrared wavelengths involved, so this type of IFB displays a higher thermal conductivity compared with the cast. Conversely, the microporous structure of the cast IFB, with its small pore sizes, is much more efficient at interfering with energy transfer at infrared wavelengths, so this type of IFB displays low thermal conductivity. This is why the microstructure of the cast IFB provides superior insulation compared to the cement IFB and is the most common form of IFB commercially available.
Another important consequence of the energy savings achieved using the lower thermal-conductivity IFB is the reduction in CO2 emissions. Using the cast IFB instead of the cement IFB reduces the environmental impact of running the kiln.
The choice of IFB in the kiln lining will also impact other practical aspects of using the kiln in a production environment. Selecting the cast IFB rather than the cement IFB will allow faster heating and cooling rates in the kiln, because the lower density cast IFB has a lower thermal mass. This effect was observed in testing and validated by CeraMaterials’ customers in the field.
Shapes
There are different standard shapes and sizes for IFBs depending on the application.
Rectangular formats (standard squares/straights) are most common, being used for straight walls and floors. Standard sizes are 9 x 4.5 x 2.5″ and 9″x 4.5 x 3″, with splits at half the thicknesses.
There are also a series of standard arch bricks which include various side and arches, wedges, keys, side and end skews, side and end feathers, and splits. These standard shapes are used for arches and round wall shapes and can be computer modeled to fit any curved space and archways fairly exactly. Figures 1 and 2 demonstrate these various standard shapes.

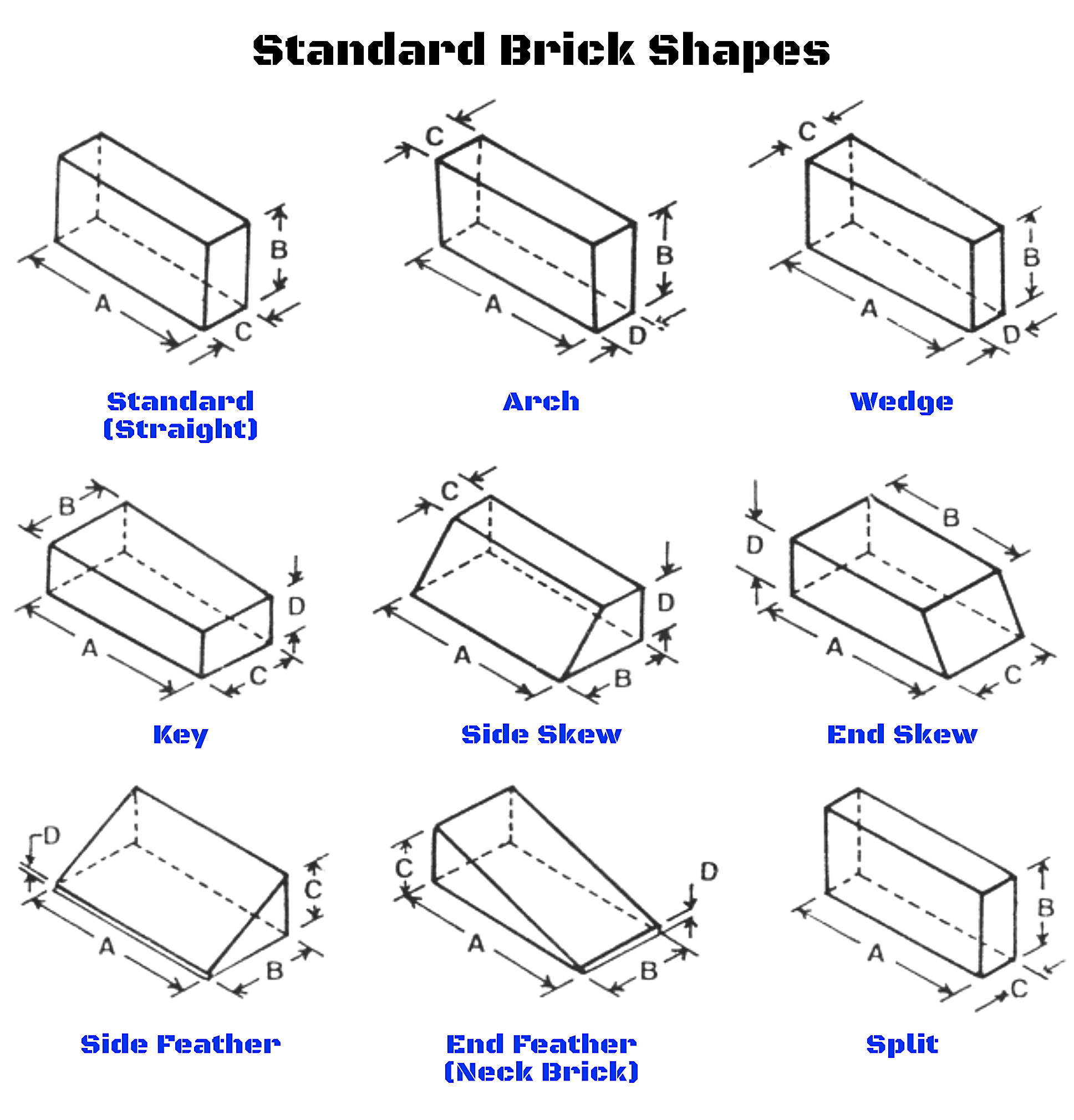
In the United States, straights are readily available in groups 23 through 32 from stock in 2.5- and 3-inch thicknesses from several suppliers, stocked, and shipped in pallets (Figure 4).


For most shapes, angled and arches, these IFBs are rarely stocked, instead being cut and ground to size per order by several suppliers with fast turn arounds. There are still thousands of kilns, furnaces, melters, and heat-treat ovens made of IFBs that need rebuilds and refurbishing due to wear, damage and overuse. In recent years, new IFB kiln fabrication has been more limited to smaller specialty kilns with ceramic fiber insulation replacing some of the IFB applications in larger furnaces.