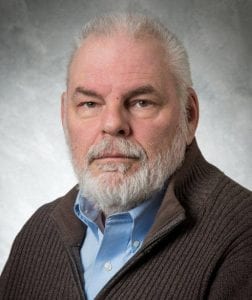
In this column, I will discuss the effect of cooling rate after tempering.
Introduction
During heat treatment of steel, the process is generally austenitize, quench, and temper (Figure 1). The heat-up rate during austenization is typically not critical as long it does not cause any distortion from unequal heating. In earlier articles, I have shown that the quench rate is critical to achieve the desired mechanical properties, and a microstructure of nearly 100 percent martensite.
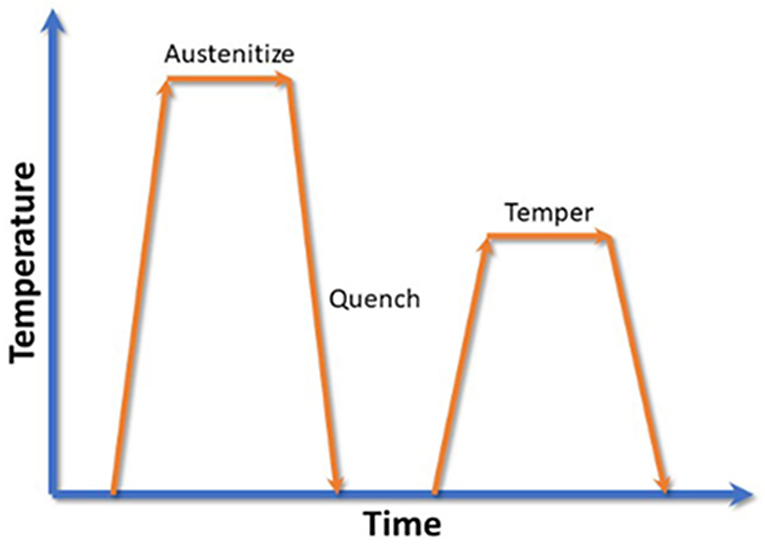
The tempering operation is critical to transform the martensite to tempered martensite, and to relieve residual stresses. There are several variables that affect microstructure and the mechanical properties of a tempered steel:
- The composition of the steel, including alloying elements.
- Tempering temperature.
- Tempering time.
- Cooling rate from the tempering temperature.
In this article, I am strictly talking about the cooling rate from the tempering temperature.
Tempering temperature is obviously the most important variable. The tempering temperature controls the size and distribution of the carbides formed. In general, the higher the temperature, the softer the part. In alloy steels, besides the Fe3C carbides found in carbon steels, additional alloy carbides will be formed. This makes alloy steels harder than plain carbon steels at the same tempering temperature.
The toughness of a steel is improved by higher tempering temperatures. However, a drop in toughness occurs in the 230 to 370°C (450-700°F) temperature range. This temperature range is the “blue brittleness” range [1], where a characteristic blue-tinted oxide forms on the part during tempering.
Temper embrittlement occurs when the steel is tempered between 450 and 600°C (840 and 1,110°F). The cause of temper embrittlement is thought to be associated with impurity segregation at the grain boundaries. These impurities are often arsenic, antimony, and phosphorus [2]. Manganese and chromium can also migrate to grain boundaries along prior austenite grain boundaries, resulting in embrittlement [3]. Fracture morphology is often intergranular.
Cooling Rate after Tempering
Looking at a typical iron-carbon phase diagram, in the temperature region commonly used for tempering, there are no changes in microstructure. It is a two-phase region of strictly ferrite and carbide. When you look at a Continuous-Cooling-Transformation diagram for steels, it only shows the reactions for transforming to martensite, bainite, or pearlite. There is no region for transformation to tempered martensite. This implies that there is no change in microstructure regardless of cooling rate after tempering.
Testing of samples cooled at different rates after tempering showed no difference in the tensile strength of the parts. However, toughness, measured by impact testing (Charpy or Izod), is decreased when cooled slowly through the temperature range of 600 to 450°C (1,110 to 840°F). For steels containing chromium and other carbide-forming elements, this is especially true [2]. Other measurements of ductility, such as elongation or reduction in area, may also be reduced when slow cooling through this temperature range. This is shown in Figure 2.
Often, temper embrittlement can be reversed by retempering above the embrittlement temperature (>600°C or 1,110°F), followed by rapid cooling to remove the embrittlement effect [7]. The time required depends on the quantity of impurities present [8].
Conclusions
In this article, we have described the beneficial effect of rapid cooling from elevated temperature tempering. Tempering in the temperature range of 450 and 600°C (840 and 1,110°F), or when slow cooling (furnace or air-cooling) through this temperature range, can allow impurities such as phosphorus, antimony, or tin, to segregate to grain boundaries. Strong carbide formers, such as chromium, can precipitate at grain boundaries, reducing toughness. Rapid cooling through this temperature range, while not affecting tensile properties, can substantially improve toughness, elongation, and reduction in area.
Should you have any comments regarding this article, or any suggestions for future articles, please contact the author or editor.
References
- I. E. Dolzhenkov , “The nature of blue brittleness of steel,” Metal Science and Heat Treatment, vol. 13, pp. 220-224, 1971.
- ASM International, “Tempering,” in Steel Heat Treating Fundamentals and Processes, vol. 4A, J. L. Dosset and G. E. Totten, Eds., Materials Park, OH, ASM International, 2013, pp. 327-351.
- G. Krauss, Steels — Processing, Structure, and Performance, 2nd ed., Metals Park, OH: ASM International, 2015.
- M. A. Grossman and E. C. Bain, Principles of Heat Treatment, 5th Edition ed., Cleveland, OH: American Society for Metals, 1964.
- Z. Cai, L. Wang, J. Pan, S. Qiao, X. Huo, P. Wang and X. Liu, “Effect of the tempering cooling rate on the toughness of bainite welds,” J Tsinghua Univ (Sci & Technol), vol. 55, no. 10, pp. 1045-1050, 2015.
- G. Krauss and C. J. McMahon, “Low Toughness and Embrittlement Phenomena in Steels,” in Martensite, G. B. Olsen and W. S. Owen, Eds., Materials Park, OH: ASM International, 1991, pp. 295-321.
- I. Olefjord, “Temper Embrittlement, Review #231,” Int. Metall. Rev., vol. 23, pp. 149-175, 1978.
- T. Inoue, K. Yamamoto and S. Sekiguchi, “Grain Boundary Segregations of Phosphorus and Manganese in Low Carbon Manganese Steels,” Transactions of the Iron and Steel Institute of Japan, vol. 14, no. 5, pp. 372-374, 1974.