
Non-ferrous alloys have caught my interest throughout my career, particularly aluminum. From its resistance to corrosion to its sensitivity at elevated temperatures, it is an interesting material. Moreover, it changed the aerospace and other industries due to the material properties of aluminum.
Following are some of the more interesting aspects of aluminum.
A History
Aluminum is not a rare metal; it constitutes around eight percent of the Earth’s crust. Its late appearance on the metals scene can be attributed to its strong attraction to oxygen. It binds itself tightly in chemical combinations that are extremely difficult to break down from composites such as clays, schists, and mica (types of minerals). The oldest known of these composites — for centuries referred to as “earths” — is alum, which was used in China 3,800 years ago in the preparation of medicines and tanning. In the centuries after that, “earths” took on the name “pure clay,” then “alumina,” from which the name “aluminum” comes.
Slow Development
In 1807, five new metals were discovered by British chemist Humphrey Davy (1778-1829). One of these five was aluminum. Due to aluminum’s strong attraction to oxygen, he was unable to isolate the aluminum using an electric arc. In Paris, 1854, Henri Sainte-Clair Deville (1818-1881) (Figure 1) continued experiments to isolate aluminum. He was convinced electrolysis was the most efficient way to produce high-volume pure aluminum. He began experiments using electrolysis, but soon found the cost of battery power was too expensive and had to end his experiments.
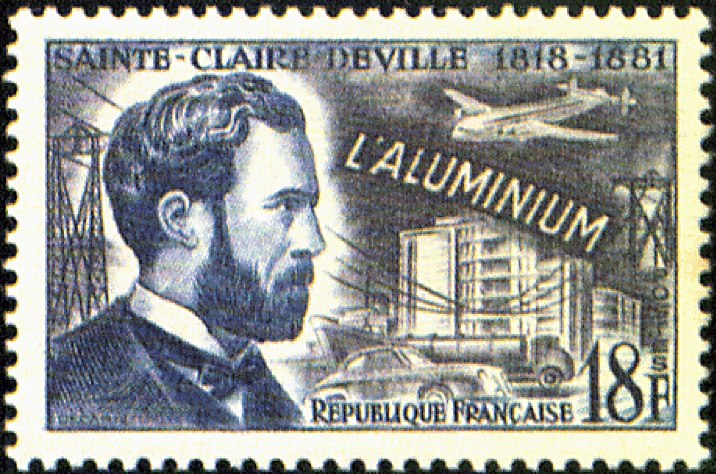
Beginning of the Aluminum Industry
In 1860, Sainte-Clair Deville partnered with Henry Merle, the founder of the PCAC, which produced soda. This plant had the necessary raw materials (bauxite) in place to launch aluminum production. Sainte-Clair Deville was the first to introduce bauxite (15-30 percent aluminum) into the process. Bauxite gets its name from the village Baux-de-Provence, where it was discovered in 1821.
Due to the red color of bauxite, it was originally thought that it would be good for the steel industry. Analysis showed that it was low in iron content but had high alumina content. Forty years later it would be the raw material of the aluminum industry. In 1887, Karl Bayer (1847-1904) patented a process that would be adopted in 1893. The first refinery to purchase the license was Gardanne in the Bouches-du-Rhone region of France. The process uses bauxite mixed with caustic soda, dissolved by heating at high pressure to 250°C (482°F) in autoclaves, then decanted and filtered. The hydrated aluminum oxide is separated from the cooled filtrate and calcined (dried) to obtain an alumina suitable for electrolytic process (Figure 2). This process was adopted in the 1950s following technological advances, particularly the development of an automated chain of operations.
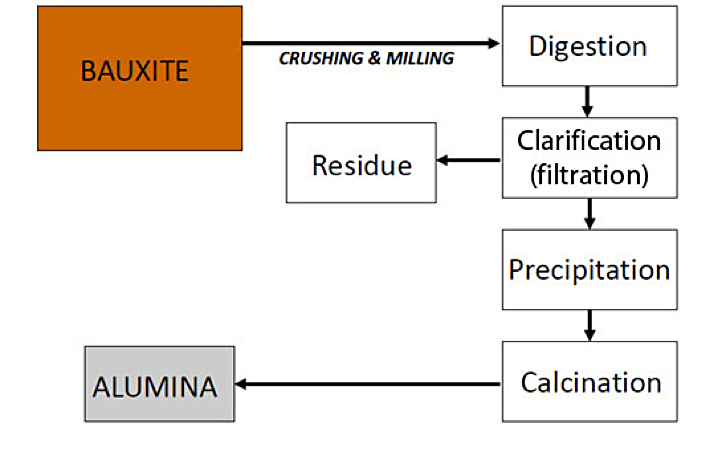
Mining Resources and Control
It takes four tons of bauxite to produce two tons of alumina, which is needed to produce one ton of aluminum. Controlling mining resources was of primary concern in the aluminum industry. Small-scale production sites were opened in various areas that were subsequently bought by larger entities in the highly competitive industry. France held the top position in bauxite mining until the end of WWI. Today, known reserves are estimated at more than 20 billion tons, representing three centuries of production at the current rate. The principal production zones are Australia, Africa, China, India and subtropical America (Figure 3). The last French mine closed in 1991.

Alumina to Aluminum – Electrolysis
The aluminum oxide is melted and electrolyzed. The anode is made of graphite, a form of carbon. Oxygen ions move to the anode where they’re converted to oxygen. The anodes are gradually worn away by oxidation. The cathode is also made of graphite. Molten aluminum is produced there. The process requires a lot of electrical energy, which is one reason why aluminum is more expensive than steel (Figure 4).
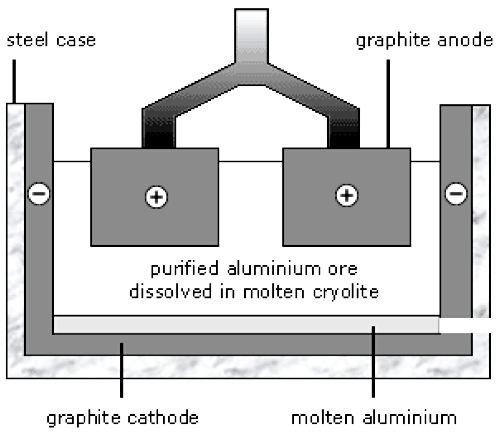
Heat Treatment of Aluminum
Aluminum is typically classified as two types: heat treatable and non-heat treatable. Following this, alloys are then classified in temper codes designated by the Aluminum Association. By definition, heat treatable aluminum alloys are those that can be strengthened by a suitable thermal process for that particular material. Let’s use A356 as an example. Solubility of the alloy elements within A356.0 are directly related to temperature, although alloy element wt% is a critical factor.
In general, solution heat treating takes advantage of the precipitation hardening reaction. Its objective is to take into solid solution the maximum practical amount of the soluble hardening elements in the alloy. This process also consists of soaking the alloy at a temperature sufficiently high and for a long enough time to achieve a nearly homogeneous solid solution. Keeping with our example, solution heat treating of A356 castings produces the following effects: it dissolves Mg2Si, homogenizes the casting, and changes the morphology of eutectic silicon. One of the most important aspects of this is the dissolution of Mg2Si. Under equilibrium conditions, the solubility of the precipitating Mg2Si phase decreases with temperature. A casting removed from the mold at 800°F will have approximately 0.3 percent Mg in solution. At 700°F, approximately 0.2 percent Mg will be in solution. This means that a drop of 100°F will result in a loss of approximately one third of the strength available from dissolved magnesium.
To obtain the maximum concentration of magnesium and silicon, the solution temperature must be as close as possible to the eutectic temperature, ideally 10° – 15°F below the eutectic temperature.
Control of temperature is critical. If the melting point is exceeded, incipient melting (localized melting at the grain boundary) may occur and mechanical properties may suffer. This condition is only detectable by metallographic examination and is irreversible. Hence, most aluminum solution heat-treating furnaces must have a temperature uniformity of ±10°F.
Solution heat-treating time is critical to ensuring the mechanical properties are conforming.
As discussed earlier, this is due to the vital role that the eutectic silicon morphology plays in obtaining satisfactory mechanical properties (Figure 5). In short, silicon particles are broken down into smaller fragments and gradually spheroidized (in physical metallurgy, a process consisting of the transition of excess-phase crystals into a globular — spheroidal — form). Prolonged solution time may, in turn, lead to coarsening silicon particles.

Quenching
The purpose of quenching is to keep the Mg2Si from forming precipitates. If done correctly, this yields maximum strength and good elongation in castings. Two variables affect the rate of cooling: quench delay and quench medium and its respective temperature.
Verification of Properties
Verification of properties is typically done in two ways — hardness testing and conductivity testing. This can be dependent on the material and thickness as well as state (i.e. casting, machined bar, etc.). Tensile testing may also be used depending on material process specifications.
Summary
Several materials stand out, depending on an engineer’s interest. Nonferrous alloys are particularly interesting due to their wide range of uses and sensitivities. I hope this article allowed readers to appreciate the different aspects of aluminum.
References
- Aluminum – Light at Heart, Ivan Frinberg, 2003.
- Metallurgy for Engineers, R.C. Rollason, 4th edition, 1973.
- ASM Handbook, Heat Treating of Nonferrous Alloys, Volume 4E, 2016.
- ASM Metals Reference Book, 3rd Edition, 1993.
- ASM Metals Handbook, Desk Edition, 2nd Edition, 1998.