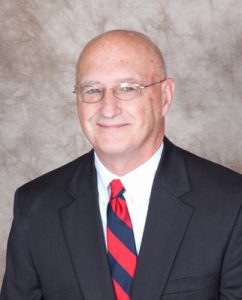
Having worked in the heat-treating industry for 58 years, I agreed in January 2011 to write a monthly column about all things heat-treating for Gear Solutions magazine. A few years later, sibling Thermal Processing began focusing exclusively on heat treating and I moved to that publication. I accepted the task for two reasons: I wanted to give back in a sense — to share my experience, and perhaps entice a new generation of participation in the industry.
But I also felt that to achieve those objectives, I needed to provide a very brief narrative on how the industry, primarily carburizing and case hardening, got to where we are today.
Following the above philosophy, a question recently came up at lunch that prompted the following discourse:
As most interested parties know, vacuum carburizing (VC) has been around for decades. But what may not be so apparent is why it became a viable process in the first place. Metals Handbook Volume 2 (copyright 1964) doesn’t even mention VC — only that vacuum has application for brazing and other processes that must be free from oxygen contamination. But how did it get its start? It likely was an experiment in a bell jar gone wrong somewhere in Europe. That isn’t really the point. What is important is that once those experiments continued through the years, progress really began in the late ’60s when it was discovered that carbon using propane in a vacuum partial pressure could be added to steel and the case depth increased more so than with endothermic gas — which was the only mainstream commercial carburizing process at the time.

What has proceeded ever since is how to control and predict the surface carbon and case depth that infrared, dew point, and now the oxygen probe could do for endo carburizing. Since to date no one has developed a similar device such as the oxygen probe for VC, we’ve no alternative but to rely on one of two methods to provide a starting point for predictive modeling software: the iron-carbon equilibrium diagram, or running an actual sample and analyzing its diffusion profile. There have been attempts by any number of entities to develop an oxygen probe or shim-test like device but to my knowledge none have so far been successful.
As the search continues for that elusive device, an even better method for predicting and controlling endo carburizing is still occupying the minds of the heat-treating community.
As the pursuit for control perfection continues, and once we know how to predict the outcome with unmatched precision, the carbon must still be able to enter the steel being carburized via LPC or endo or whatever means evolves.
That brings me to the next conundrum, namely carburizing temperature.
Two conflicting viewpoints seem to be the barrier or wall that separates the pro high-temp energy savers vs. those on the con high-temp side who fear the reduction of mechanical properties. Many of those on the con side are comprised of the aerospace industry and producers of high strength components such as fasteners like bolts. On the aerospace side, both LPC and endo carburizing are used depending on the application. There remains, I believe, a subtle fear of LPC in extremely critical parts, notably very large components, especially gears that are press quenched where LPC is not applicable. Endo carburizing is so well understood and predictable, and the equipment so robust that you’ll find seemingly antique furnaces, but well maintained, successfully producing extremely high-quality aerospace components. LPC equipment by design requires frequent and specialized maintenance where endo furnaces do not.
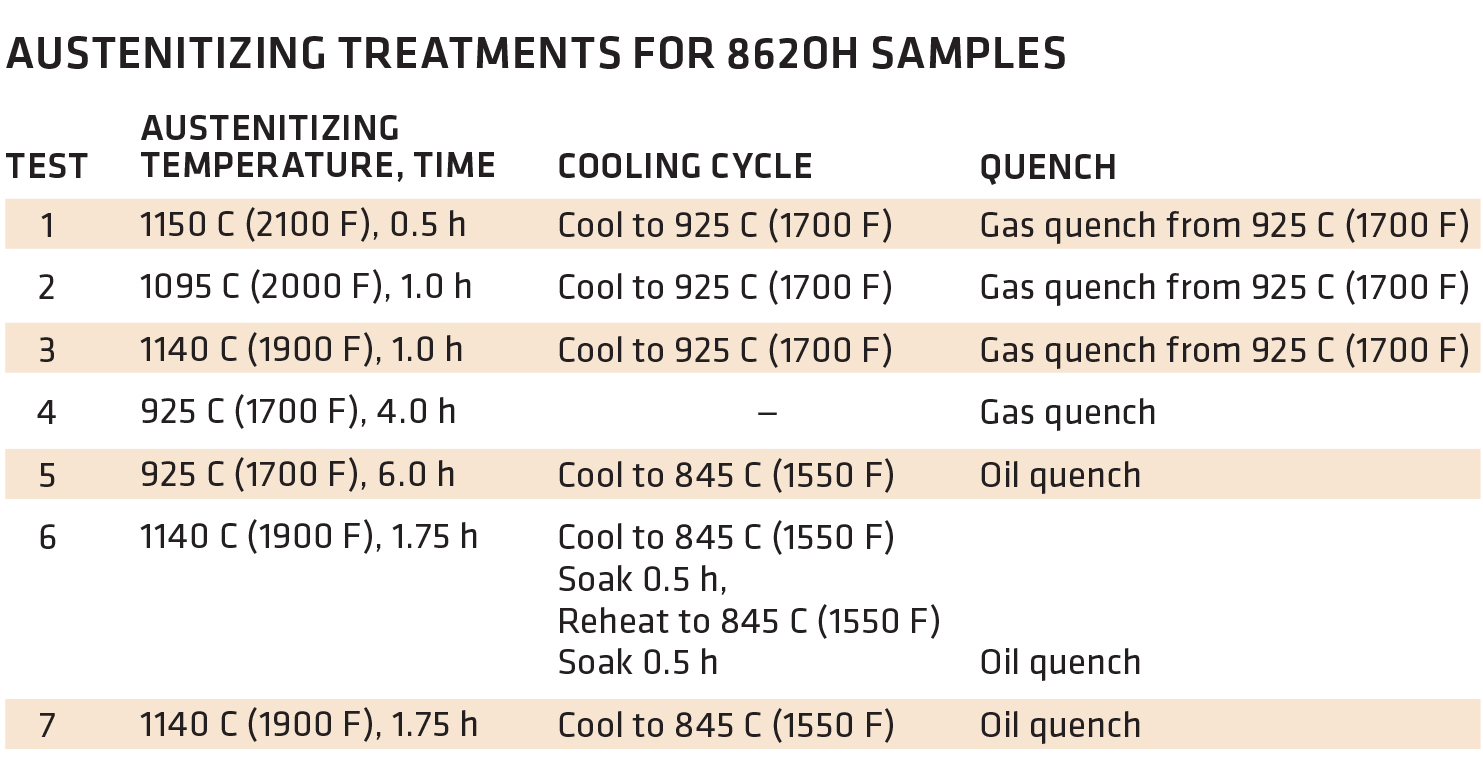
Most of the LPC and endo carburizing is conducted between 1,650°F and 1,700°F (899°C and 926°C). Vacuum furnaces can only perform LPC because they’re vacuum furnaces designed with fragile graphite and molybdenum and operate up to 2,400°F (1,315°C) so it’s in their best interest to promote high-temperature carburizing primarily above 1,850°F (1010°C). Likewise, endo gas atmosphere furnaces are also capable of carburizing at higher temperatures, however they have limitations due to the use of metallic or iron-based heat resistant nickel, chromium, cobalt alloys that are capable of operating at 1,900°F or 1,950°F (1,038°C or 1,065°C).
The temperature dilemma relates to grain size and its effect on cracking from fatigue and tensile stress. It’s well known that if the carburizing temperature is too high for the material austenitic grain growth can weaken the component. It’s not too difficult to understand, but I like to make the analogy to the concrete overpasses we encounter every day on the highway. The overpass is continuously subjected to fatigue from traffic and wind forces. To counter those forces, designers added rebar, steel bars intertwined and crisscrossed throughout the concrete structure, to stiffen and strengthen the entire structure. Without rebar or with fewer numbers of steel rods, large unreinforced sections of concrete would begin to form small fatigue cracks — and without rebar, the cracks can continue uninterrupted.
In steel, a similar process takes place. The finer the grain size, the greater resistance to fatigue. Like rebar, the individual grains that are formed in the hot swaging of steel bar or hot rolling of plate, mechanically reduce the size and increase the number and orient the grains in the direction or rolling. If each post heat-treat process such as annealing, normalizing, and carburizing is completed within accepted limits, that original small grain size will be retained. However, if overheating such in carburizing in order to save time is conducted, mechanical properties can be sacrificed. The question becomes what is overheating? There’s no complete definitive answer because it depends on the alloy additions the mill adds to help resist grain growth; that’s why 1,750°F (954°C) is today the practical limit to avoid the risk.
Now I’m going to be my own devil’s advocate:

There are ways to carburize at higher temperatures without sacrificing properties. I’m referencing an article published in Metal Progress, a publication of The American Society for Metals, called “Effects of High-Temperature Austenitizing on AISI 8620H,” written by James G. Conybear and Wallace (Jack) Titus (November 1977 Vol. 112, No. 6). Jim was the primary author with yours truly conducting the tests on the two-chamber oil quench vacuum furnace, Figure 1, and performing the Charpy impact and tensile tests, Figure 2. The article set out to investigate if the time corresponding to specific carburized case depths at elevated temperatures for 8620H steel had any detrimental effects on mechanical properties. We didn’t carburize the material but held it at various temperatures to affect the core properties (Table 1). I won’t present the entire text but summarize the results with these three figures. Bottom line, at least for this 8620H study, processing at elevated temperature had no negative effect on the properties evaluated. For those interested, the entire article can be accessed at tinyurl.com/yxvftfbc.
Finally, since major steel producers are developing new micro alloyed carburizing grade steels that can retain critical room temperature properties, several furnace manufacturers including AFCH have and are developing hot zones to take advantage of the new materials.