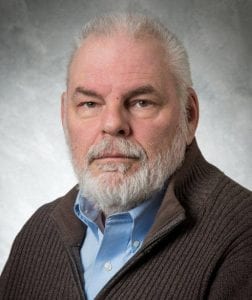
In this short column, we will discuss quenching with water. As a quenching medium, plain water approaches the maximum cooling rate attainable in a liquid. Other advantages include no health hazards, readily disposed, no fire hazard, and readily available. It is also inexpensive compared to other quenchants. Water is used whenever the drastic quench will not cause cracking or excessive distortion. It is widely used for quenching non-ferrous alloys, austenitic stainless steels, and low hardenability carbon steels.
One disadvantage of plain water as a quenchant is that its rapid cooling rate persists throughout the lower temperature range, in which distortion or cracking is likely to occur. Water has a high quenching power (heat transfer coefficient) due to the high specific heat of vaporization and high specific heat capacity. The thermal conductivity is very small compared to most metals. The boiling temperature of water (100°C) is also very low compared to the transformation temperature of martensite for most steels. Because of this, the maximum heat transfer of water occurs at low temperatures. This can contribute to cracking excessive distortion of heat-treated parts.
Consequently, water usually is restricted to the quenching of simple, symmetrical parts made of low hardenability steels. Another disadvantage of using plain water is that its vapor blanket stage may be prolonged, especially at elevated temperatures. This stable vapor phase encourages vapor entrapment.
Local temperature may be increased, resulting in uneven hardness and unfavorable distribution of stress. This may cause distortion, cracking, or soft spots. Water quenched steel parts may rust unless immediately treated with a rust preventive.
Temperature
The temperature at which water boils (and the maximum cooling rate or maximum heat transfer) occurs within the range of most martensitic transformation temperatures for most grades of steel. Because of the low temperature of maximum heat transfer, and the persistent vapor phase, very large differences in heat transfer can occur. Vapor can persist in holes, cavities, and on surfaces with adjacent parts. Further, the very rapid cooling rate can contribute to large thermal stress, contributing to distortion in quenched parts.
If the water quenchant temperature is increased, two things occur. First, the vapor phase becomes much more pronounced and stable. Secondly, the maximum cooling rate during nucleate boiling decreases. In addition, the temperature of maximum cooling also decreases as the temperature of the water is increased. As the temperature is increased, the cooling rate and resultant thermal stresses decrease, but the transformational stresses increase. This is shown in Figure 1.
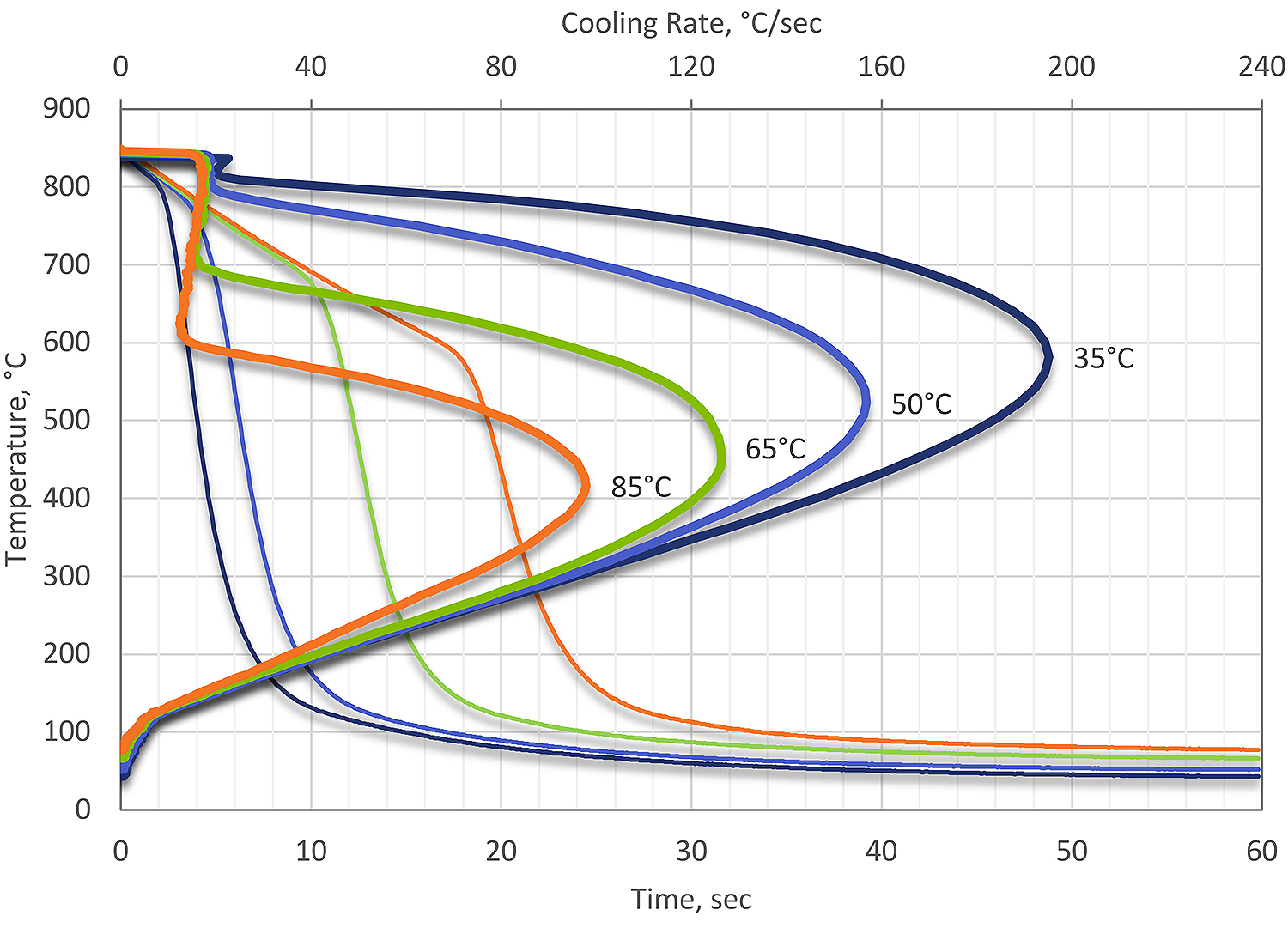
In most cases, water quenching is accomplished at room temperature (15-25°C). If the water is not well agitated, then highly variable heat transfer can occur. This is also true of non-symmetrical parts, where large differences in heat transfer can result in distortion or cracking.
At temperatures below 100°C, there is little effect on the cooling curve due to increasing temperature. For steels, there is really no benefit to increasing the water temperature during quenching. For aluminum, for large forgings, there is some benefit to using elevated water quenching. The slow quenching rate reduces the residual stress from quenching. However, the properties suffer. Much better results can be accomplished using a polymer quenchant to achieve desired properties and reduced distortion.
Agitation
Agitation is particularly important in water quenching because of the very stable vapor phase. The purpose of agitation is to wipe the vapor phase away, and to provide for uniform heat transfer on the surface of the part.
The interaction of temperature and agitation on the vapor phase is much stronger for water than in other quenchants. Therefore, water quenching is generally not used for complex shapes or blind holes. These geometrical obstructions result in a non-uniform heat transfer around the surface of the part due to a persistent vapor phase. In areas of persistent vapor phase, low hardness may result. Cracking can also occur because the local temperature may increase, which will result in shifting the maximum cooling rate to lower temperatures, increasing the transformational stresses (Figure 2).
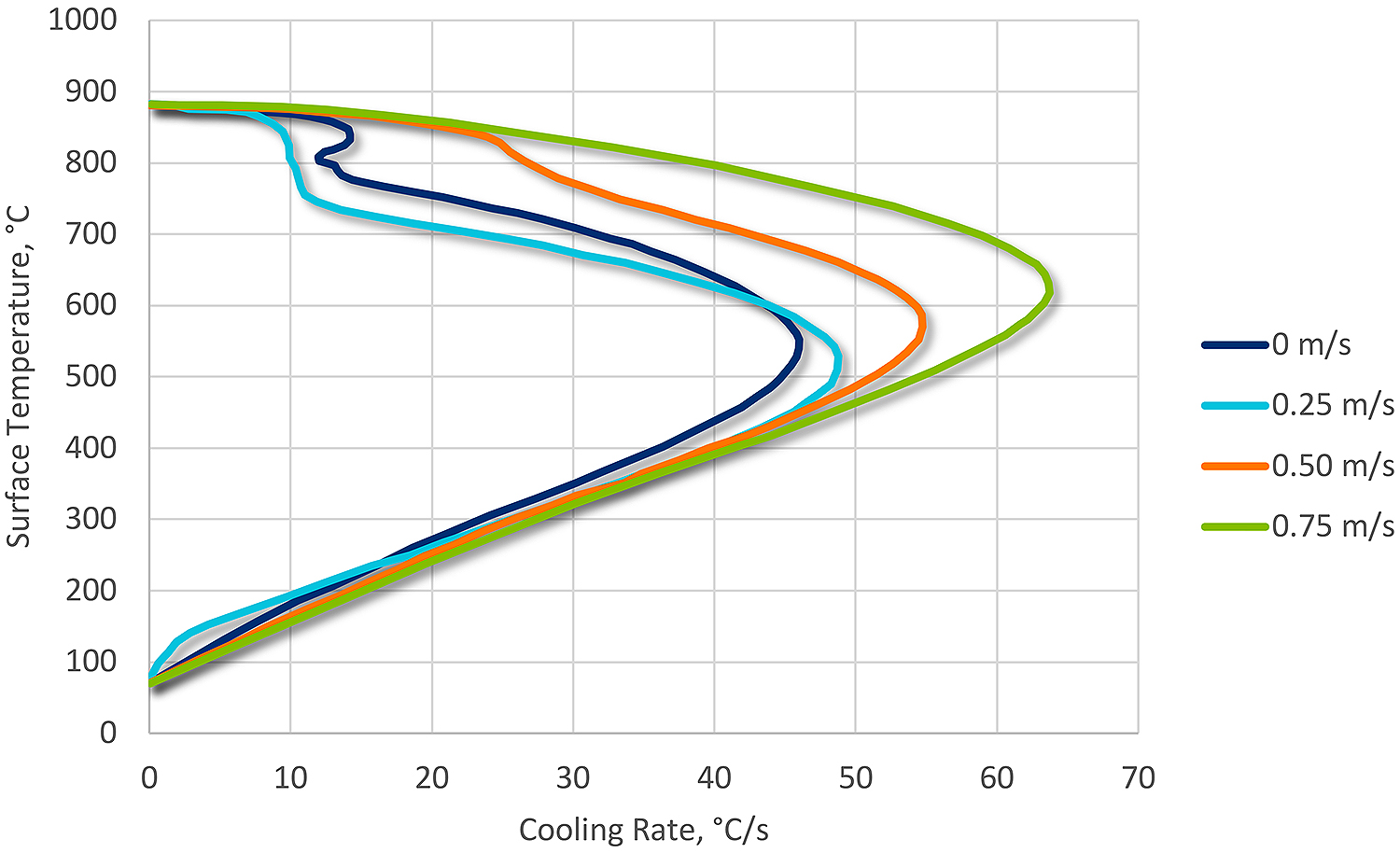
Contamination
Contamination is common in the heat-treating shop. With an open tank, it is prone to collecting dust from the shop atmosphere, pollen, and possibly bird droppings. There are also other sorts of contamination such as hydraulic fluids, oils, and scale. Some scale will separate from the part and fall to the bottom of the tank. Other fine scale can be found in the quench water itself, turning the quenchant a dull brown color.
The first group of contaminants are those that are poorly soluble in water. Solids such as soot and liquids that contain soaps, fats, and oils, form suspensions or emulsions that promote vapor phase stability. This results in an increased duration of the vapor phase with a lower temperature of nucleate boiling initiation. Oils, soaps, and fats are the most damaging. As a result, this group increases non-uniform heat transfer. This non-uniformity manifests itself as spotty hardness or increased distortion.
Dissolved gases can also behave in a similar fashion as soaps and emulsions. A saturated solution of air (or other gas) in water will come out of solution during quenching and create a very long vapor phase. This is one reason why is it is recommended to never agitate a quench tank using compressed air.
There are other sorts of inorganic contamination from the incoming water. Depending on how hard the water is, as the water evaporates from quenching or just low humidity, the inorganic content can change the quenching characteristics of the quench. These contaminants decrease the stability of the vapor phase and promote nucleate boiling. If the concentration is high, then the vapor phase may not form at all. This build-up of inorganics can increase the quench rate substantially, to the point where 60°C hard water has nearly the same quench rate as distilled water at 20°C (Figure 3).

Conclusions
To obtain reproducible results by water quenching, the temperature, agitation, and contamination must be controlled. Water at a temperature of 15 to 25°C (55 to 75°F) will provide uniform quenching speed and reproducible results. As indicated in Figure 1, the surface cooling power of water decreases rapidly as water temperature increases. Hot water has a low heat extraction because, as the boiling point is approached, the vapor phase becomes prolonged. Strong and uniform agitation will reduce the presence of the vapor phase and help promote uniform quenching.
Should you have any questions or comments regarding this column, please contact the editor or myself. Also, if there are any topics that you would like to see covered in this column, please contact the author or the editor.