
Most bearing failures can be attributed to some form of rolling contact fatigue. Many factors influence rolling contact fatigue, but two main factors are steel cleanliness and residual stress. [1] Generally, steel cleanliness has improved dramatically over the years and is becoming less of an issue. [2] Residual stress has been shown to have a significant effect on rolling contact fatigue performance and can be affected by many variables. [3-5] A variable often overlooked is partial decarburization, which can have an extremely negative impact on the residual stress profile of through hardened and case carburized components. [6] This article will examine the effects of partial decarburization on the residual stress profile of a through hardened M50 and a case carburized M50NiL bearing ring with the use of modeling.
A bearing ring with a 4-inch outer diameter was modeled using the DANTE heat treatment simulation software to show the effect of partial decarburization on residual stress. Figure 1 shows a 270° CAD section of the bearing ring. An axisymmetric model was used for the study, with the assumption that the cooling conditions act uniformly in the circumferential direction. Figure 2 shows the cross-section of the bearing ring and the line of nodes used for plots of carbon, hardness, and stress as a function of depth from the surface.
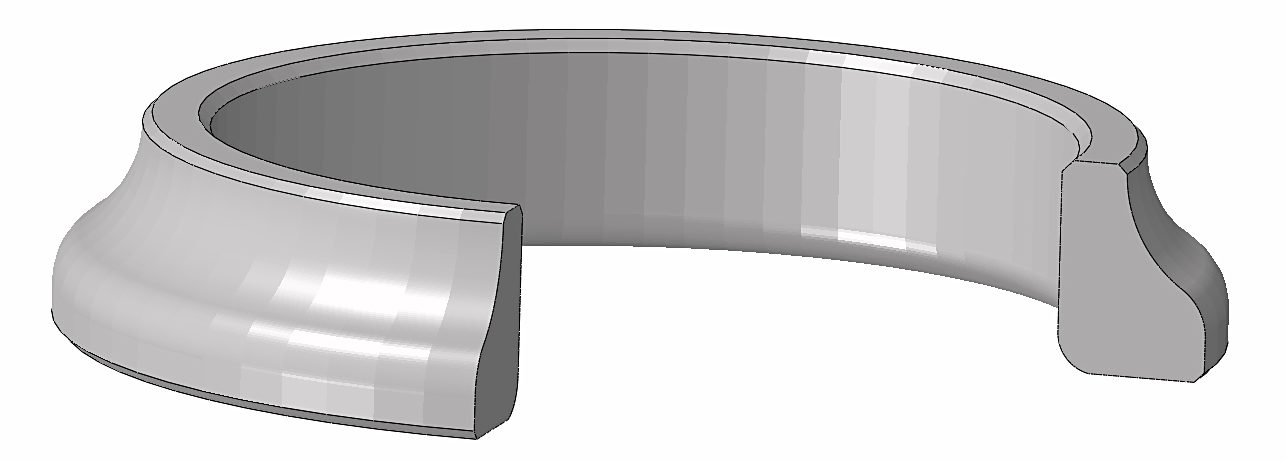
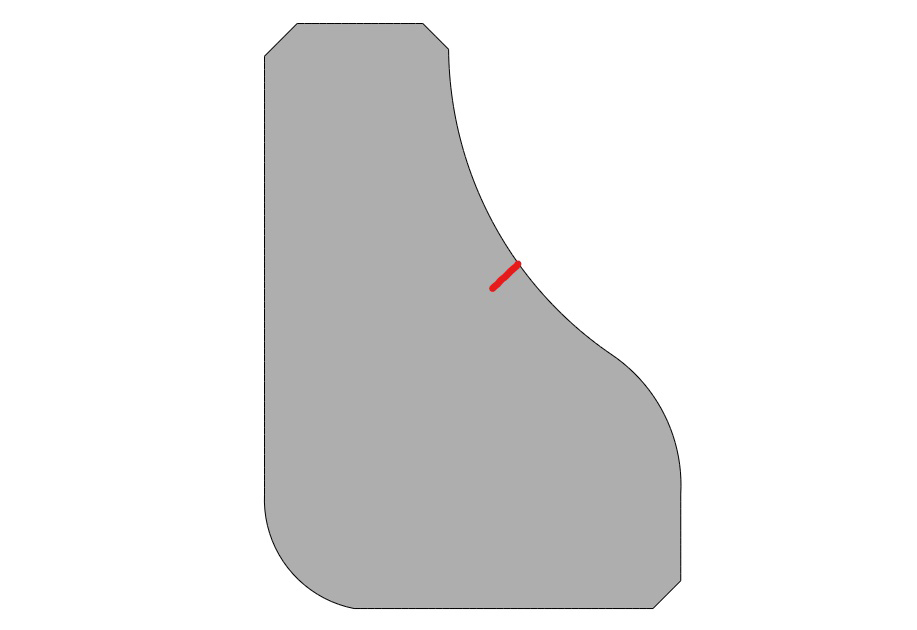
Using DANTE, the predicted residual stresses of a through hardened M50 and a case carburized M50NiL bearing were compared. It is well known that the compressive residual stresses from a case carburized bearing can substantially improve rolling contact fatigue resistance for heavily loaded bearings. [1-6] However, decarburization has the potential to alter the residual stress state at the surface of the component and affect rolling contact fatigue performance. A situation was therefore derived by which the amount of decarburization still resulted in a fully martensitic microstructure, with a hardness of approximately 60 HRC. Figure 3 compares the predicted carbon distributions for a through hardened M50 bearing and a case carburized M50NiL bearing, with and without decarburization.
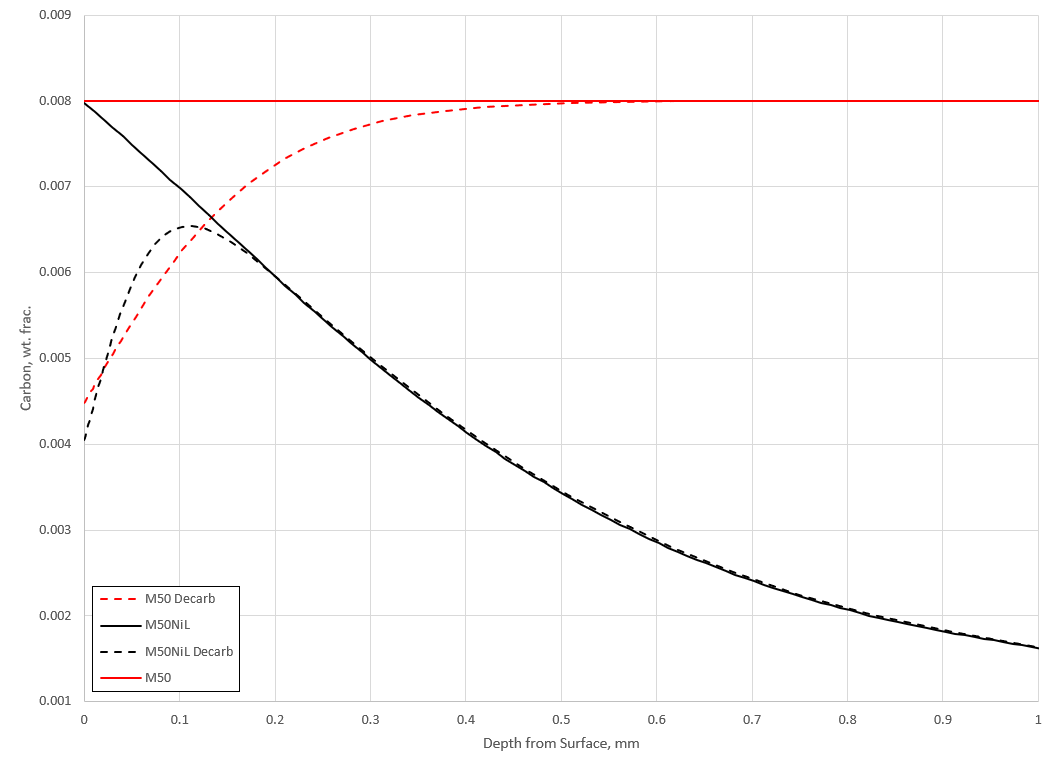
As shown in Figure 3, the amount of decarburization is approximately equal for both materials and can be classified as partial decarburization. The decarburization extends to approximately 0.1 mm, with a surface carbon value of 0.4 percent. With the carbon at 0.4 percent, the surface hardness is approximately 60 HRC for both cases, as shown in Figure 4. This means that both components would most likely be accepted by quality control. While the hardness would lead to the belief that the component would function well during rolling contact, a closer look at the surface stress state tells a different story.
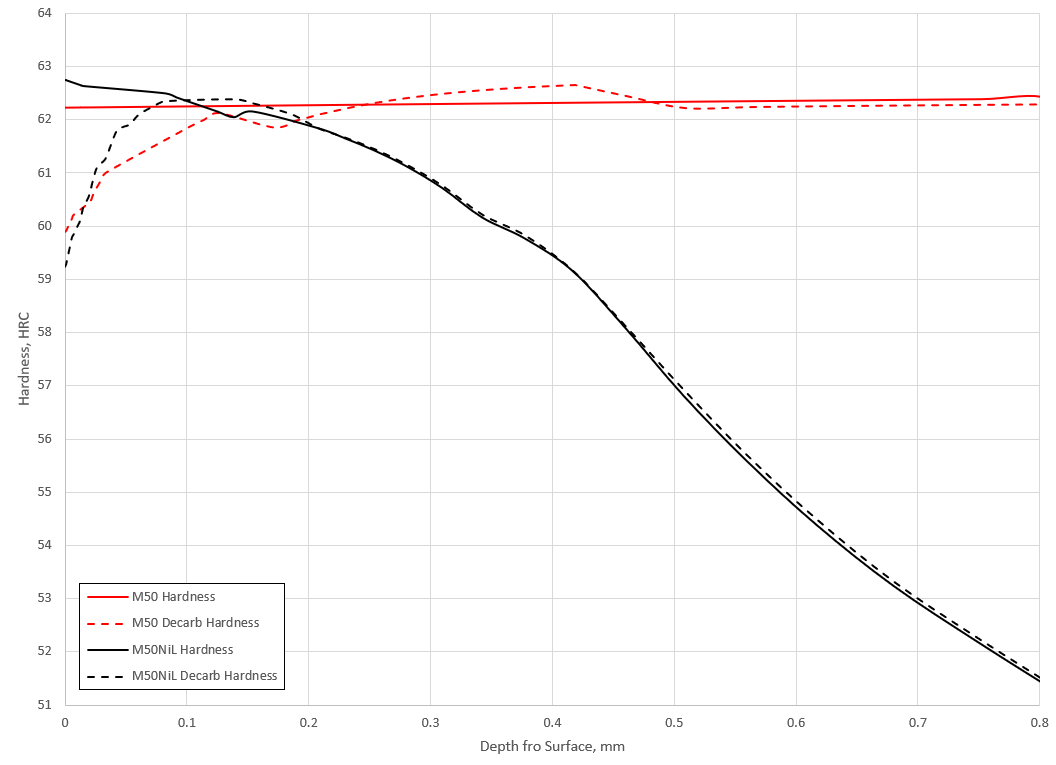
Most through hardened bearings have a slight tensile stress at the surface of the component. This is due to the phase transformation progressing from the surface to the core. Figure 5 shows the martensite and stress relationship for the M50 bearing with and without decarburization. It should be noted that the core point lies approximately
1 mm below the surface and the maximum principal stress shown corresponds to the hoop stress in the bearing’s outer raceway when that stress is tensile. Figure 5 shows that after the initial thermal contraction of the surface, followed by thermal contraction of the core point, the martensite transformation starts on the surface. This transformation causes the surface to go into compression, while the core balances the stress with tension. Approximately 15 seconds after the surface transformation, the core begins to transform to martensite. The core transformation acts to push out on the hardened surface, reducing the compression and eventually driving the surface into tension. Both conditions exhibit this behavior, but the decarburized transformation progresses much faster on the surface, such that there exists a large difference in the amount of martensite formed on the surface relative to the core. This slight difference has a very significant impact on the residual stress state.
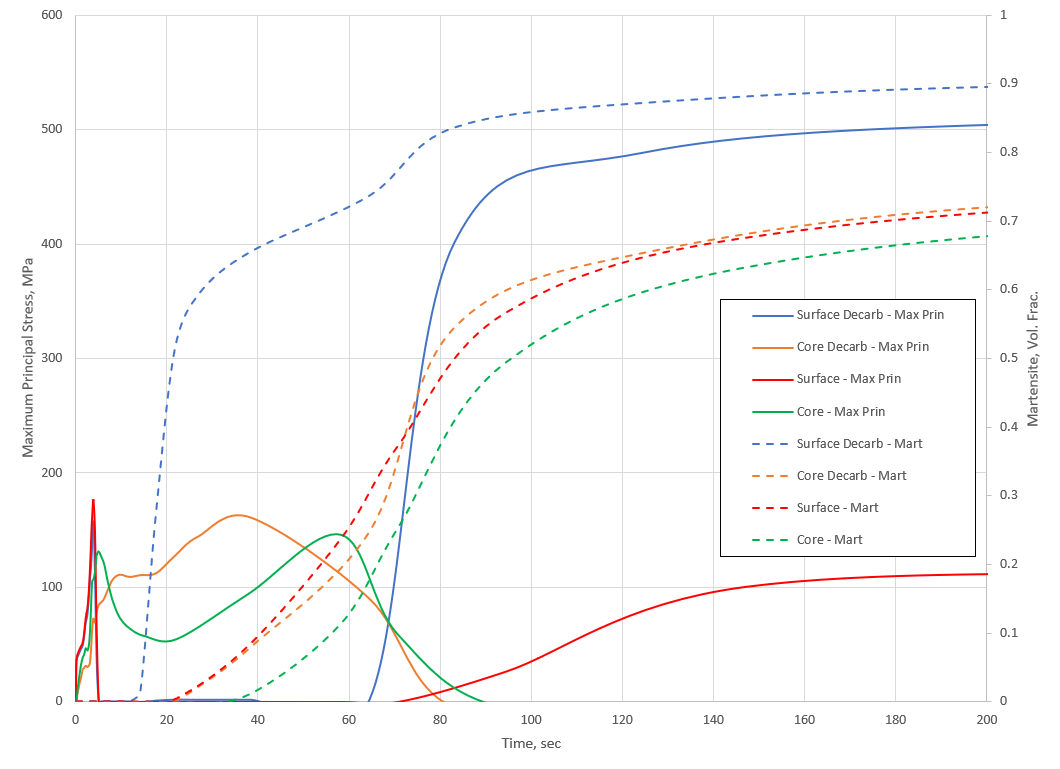
Carburized components are just the opposite, with the transformation beginning subsurface in the lower carbon region and progressing out toward the surface. This means the surface layer is the last to transform to martensite, leaving the surface in a state of compression from the volume expansion of the martensite transformation. Decarburization has the same effect with respect to martensite phase transformation timing as the through hardening behavior; the surface transforms before the subsurface material does. This behavior acts to reduce the compression generated from the carburized transformation behavior. For the amount of decarburization in this example, the surface compression of the carburized M50NiL bearing is reduced approximately 100 MPa, as shown in Figure 6. This may not be enough to seriously hurt the rolling contact fatigue performance of this bearing. However, the through hardened M50 bearing saw a 500 MPa increase in tensile stress for the hoop and axial directions. Besides being extremely detrimental to rolling contact fatigue, this level of stress may be enough to cause surface cracking if a minor surface defect is present.
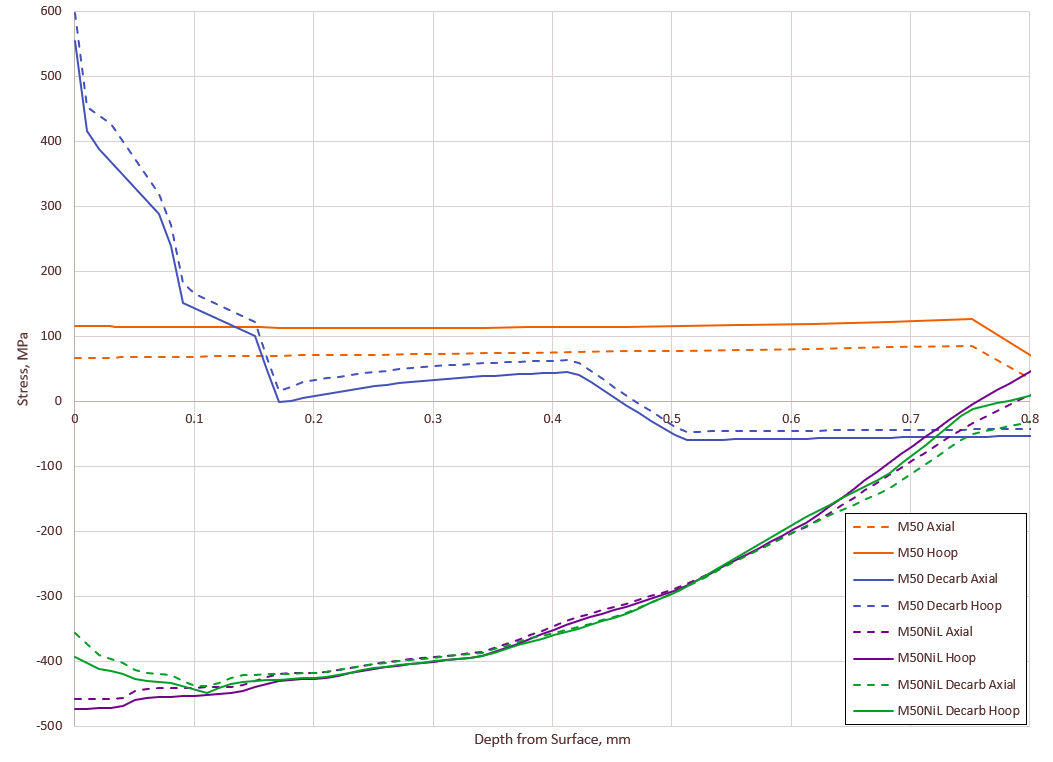
Conclusion
In summary, surface stress plays a fundamental role in rolling contact fatigue performance of bearing components. While through hardened bearings usually have some residual tensile stresses at the surface, they are generally low in magnitude. While these low magnitude tensile stresses are not helpful to performance in rolling contact, they can be easily converted to compressive by post-hardening finishing operations such as shot peening or burnishing for high-load applications. However, with mild decarburization these operations may not completely remove the tensile stresses, let alone impart compressive residual stresses. Case carburized bearings, on the other hand, use a carbon gradient to induce relatively high magnitudes of residual surface compression. Mild partial decarburization for a case-hardened bearing is far less severe, simply reducing the compressive magnitude slightly.
References
- WU Glaeser and S.J. Shaffer, “ASM Handbook, Volume 19: Fatigue and Fracture”, ASM International, pg. 331-336, 1996.
- Erwin V. Zaretsky, “Rolling Bearing Steels – A Technical and Historical Perspective”, NASA Technical Memorandum, April 2012.
- Dave Hannes and Bo Alfredsson, “Modeling of Surface Initiated Rolling Contact Fatigue Damage”, Procedia Engineering, December 2013.
- Hoa Ngan Patricia Nguyen, “Finite Element Analysis Simulation of the Effect of Induction Hardening on Rolling Contact Fatigue”, Journal of Tribology, June 2019.
- Spiridon S. Cretu, Marcelin I. Benchea, and Ovidiu S. Cretu, “Compressive Residual Stresses Effect on Fatigue Life of Rolling Bearings”, Proceedings of ASME IMECE07, November 2007.
- Geoffrey Parrish, “Carburizing: Microstructures and Properties”, ASM International, pg. 37-49, 1999.