The sintering process can improve the microstructure of Al2O3 composite ceramics and enhance their comprehensive properties, but the effects of the sintering process on Al2O3 composite ceramics are still unclear. Herein, a novel Al2O3 composite ceramic was printed using the material extrusion and photo-polymerization combined process, and the final ceramic was obtained using one-step sintering (TS) and two-step sintering technology (TSS). Based on the testing results, such as the relative density (Drel), average grain size (AGS), hardness, bending strength, and fracture toughness, TSS was suitable for the refinement of commercial Al2O3 ceramics. Moreover, the highest sintering temperature of the second step (T2) was at 1,550°C, while that of the shortest holding time (t) was at 4 hours (TSS8), which was to ensure densification before rapid grain growth. The Drel and AGS of the best ceramics obtained via TSS8 were 97.65% and 1.52 µm, respectively. Their hardness, bending strength, and fracture toughness were also enhanced, and they were affected by T2, t, and the interaction. In sum, the TSS obtained better fracture toughness and bending strength, which had great potential in the application of the additive manufacturing field.
1 Introduction
Al2O3 ceramics is one of the most common engineering materials, and its performance depends on the density and control of fine particles [1]. In the technology of obtaining dense and fine particle ceramics, fine particles in raw material will reduce the fluidity of the slurry, which has limited the form of the green body [2,3], and it will hinder the further application of the commercial Al2O3. Dense and fine-grained ceramics can be obtained via pulse plasma sintering, liquid-phase sintering, laser sintering, adding additives, etc., but the sintering process is complex, and the cost is high [4,5,6,7,8]. One-step sintering (TS) is common, economical, and easy to operate, which cannot only improve compactness through higher sintering temperatures but also promote grain growth [9,10,11]. The slightly lower sintering temperature can uniformly refine the particles and reduce their compactness [12], which limits the application of ceramic structure densification and refinement.
As ceramic fabrication technology develops, two-step sintering (TSS) is adopted to control the sintering rate and achieve the density and refinement of ceramics [13,14,15] to improve their mechanical properties [16,17]. Generally, the first step of sintering to the maximum temperature is recorded as T1. After a brief stay, it quickly cools to T2 as the second step to sintering temperature and cools down to room temperature after holding temperature for t2 [18]. The dense Al2O3 ceramics [19,20,21], BaTiO3 ceramics [22], and other ceramics [23,24] are obtained using TSS to inhibit the growth of ceramic grains.
Z. Razavi et al. [25] prepared and characterized sub-micrometer Al2O3 ceramics (grain size, 150 nm) using different TSS and discussed the effects of T1 and t2 on the densification and grain of ceramics. Compared with TS, when t2 = 60 h, T1 goes from 1,300°C to 1,150°C, the grain size in the bulk was reduced from 1.2 µm to 0.85 µm. The grain size decreased to 0.5 µm when T1 dropped to 1,250°C, and the relative density (Drel) was less than 88% at T2 = 1,100°C, indicating that temperature played a vital role for TSS, but the period of t2 was longer, and the effects on average grain size (AGS) and Drel has not been analyzed. N.J. Lóh et al. [26] used this technique to obtain the three commercial Al2O3 of different purity (92, 96, and 99 wt% of Al2O3), evaluate the effects of T2 and t2 on density, and conclude the maximum T2 (1,550°C) and minimum t2 (4 hours). Moreover, the interaction of T2 and t2 significantly affected the density of 99.7 wt% Al2O3 (particle size = 0.73 µm) [27]. However, the systematic evaluation of sintering parameters on compactness, AGS, and mechanical properties are still unclear, and the AGS is within the range of sub-micrometer (150-200 nm) and micrometer (0.73-2.16 µm), the application of TSS in multi-components of micro-nanometer particles composite ceramics has not been analyzed.
In this study, Al2O3, TiCN, and the other micro-nanometer particles were used as raw powder. The ceramic green body was fabricated via material extrusion and the photo-polymerization combined process (MEX-PPM) [28], and using two-step degreasing [29] and TSS, the final ceramic parts. The effect of the sintering process on Al2O3 composite ceramics fabricated using the MEX-PPM process was studied through Drel, AGS, mechanical properties, and microstructure measurements.
2 Materials and Methods
2.1 Materials
Al2O3 (Tuopu Metal Materials Co., Ltd., Suzhou, China) was used as the matrix material, TiCN as the additive, MgO as the sintering additive, Ni and Mo as the metal binder (Tuopu Metal Materials Co., Ltd., Suzhou, China), 0.15 wt% oleic acid (OA) as the surfactant [28], and 1,6-hexanediol diacrylate (HDDA) (Changxing Chemical Co., Ltd., Shanghai, China) as the prepolymer solution. Diphenyl (2,4,6-trimethylbenzoyl)-phosphate oxide (TPO) (BASF GmbH, Shanghai, China) was used as a photoinitiator. The properties and content of raw materials are listed in Table 1.
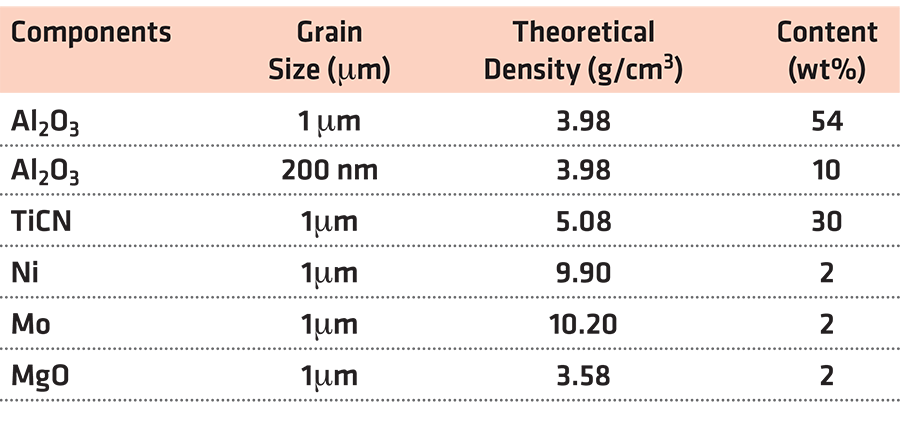
2.2 Preparation Process
The preparation of ceramic slurry for the MEX-PPM process can be divided into three steps: modifying ceramic powder, preparing prepolymer solution, and mixing slurry. Firstly, the ceramic powder was added to deionized water containing OA, mixed evenly, and dried to obtain the modified powder. Then, the modified ceramic powder was added into the HDDA prepolymer solution with TPO and milled for 4 hours. Finally, the milled slurry was subjected to ultrasonic vibration to eliminate bubbles in the slurry, and the available ceramic slurry was obtained.
In the process of printing ceramic bulk using the MEX-PPM process, the ceramic slurry is extruded through the round nozzle and deposited on the workbench, which receives UV light radiation to maintain the shape and prevents the collapse and deformation of the deposited slurry caused by gravity [31]. The round nozzle and UV light source are fixed on the equipment. The 3D printing software slices the parts to generate a G-code, drives the workbench to move, and obtains the final ceramic bulk through the layer-by-layer deposition of slurry and UV light curing. The schematic diagram of the MEX-PPM process is shown in Figure 1.
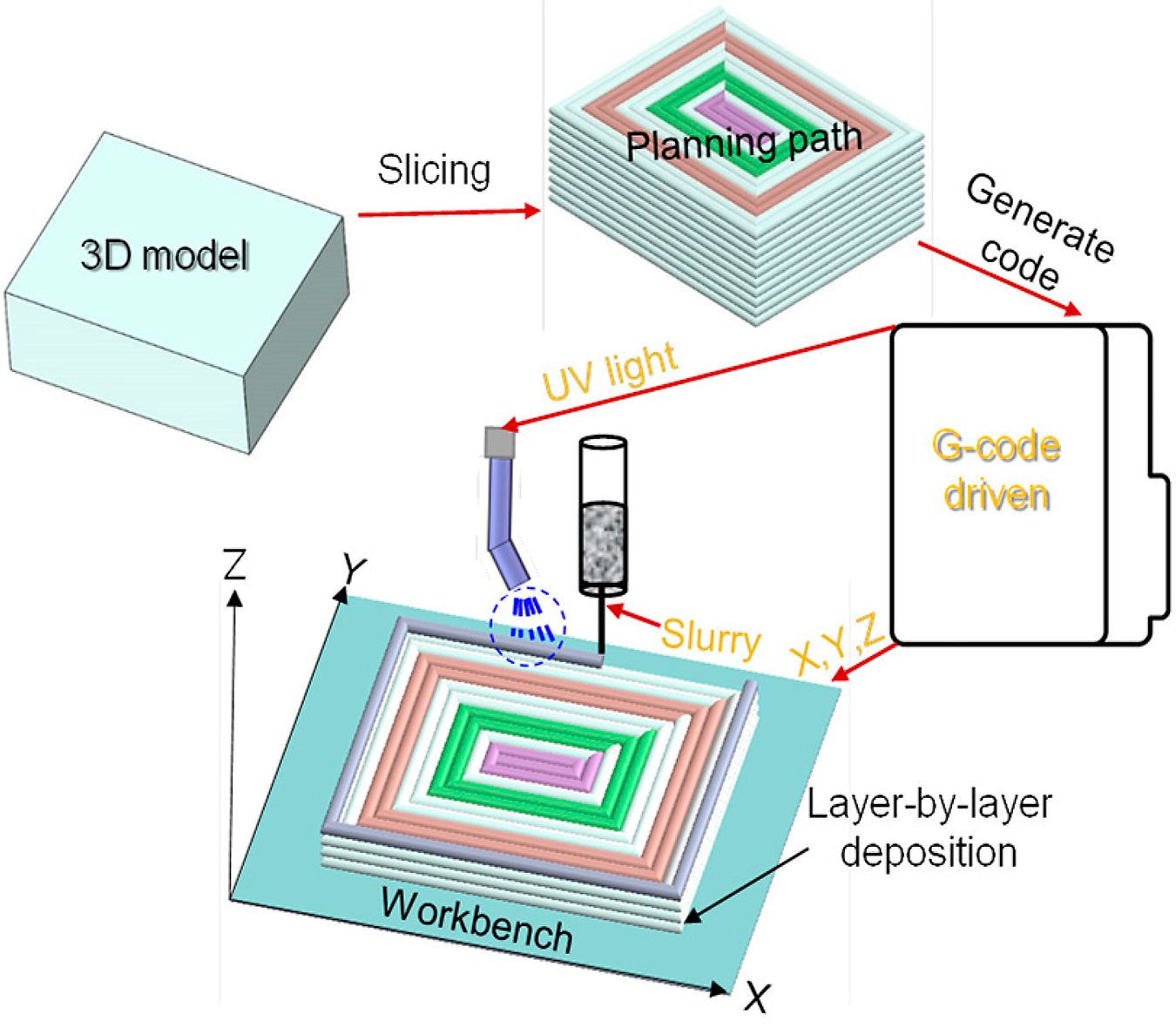
The above MEX-PPM process was used to print the dense ceramic bulk at a printing speed of 5 mm/s and a radiation energy of 20 J/cm3 at room temperature and obtain the final ceramic parts through degreasing and sintering technology.
2.3 Degreasing and Sintering Process
2.3.1 Degreasing Process
This work adopted a two-step degreasing (TSD) with a controllable pyrolysis rate [29] to remove the organic binder HDDA in the ceramic bulk; the TSD process is shown in Figure 2.
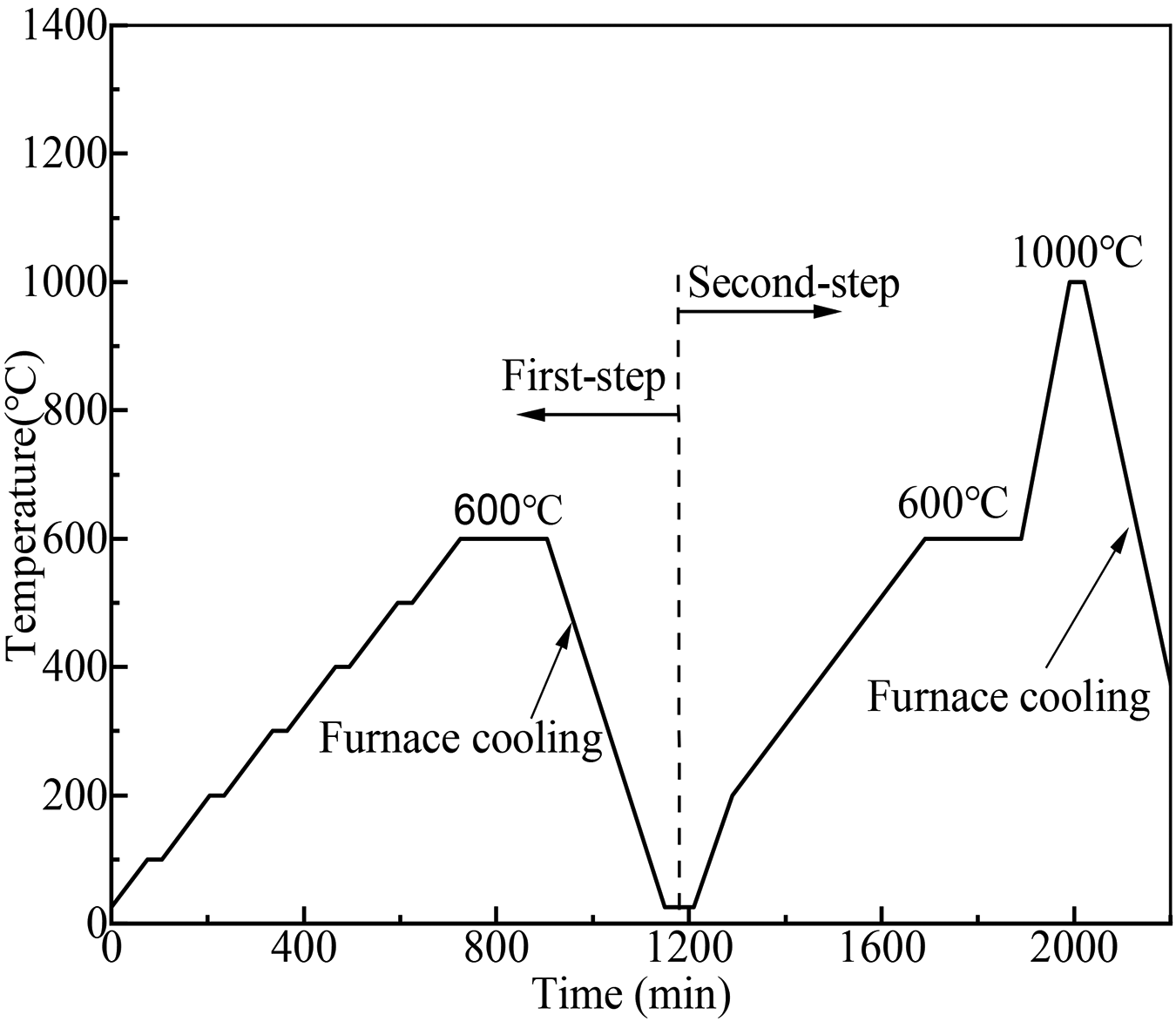
Combined with the experimental conditions, the first step of degreasing in this study was carried out in a tubular furnace (GSL-1700X, Hefei Kejing Material Technology Co., Ltd., Hefei, China) at the rate of 1°C/min. The temperature was held for 30 minutes every 100°C increased, hold for 180 minutes when it reached 600°C, and then the furnace was then cooled to room temperature. The second step of degreasing was carried out in an air furnace, which was heated to 200°C, 600°C (holding for 200 minutes), and 1,000°C (holding for 30 minutes) at 2°C/min, 1°C/min, and 4°C/min, respectively, and then the furnace was cooled to room temperature to complete the whole degreasing process.
2.3.2 Sintering Process
After degreased Al2O3 composite ceramic adopts the traditional TS and the designed TSS to obtain the final ceramic parts, the process of TS and TSS is shown in Figure 3.
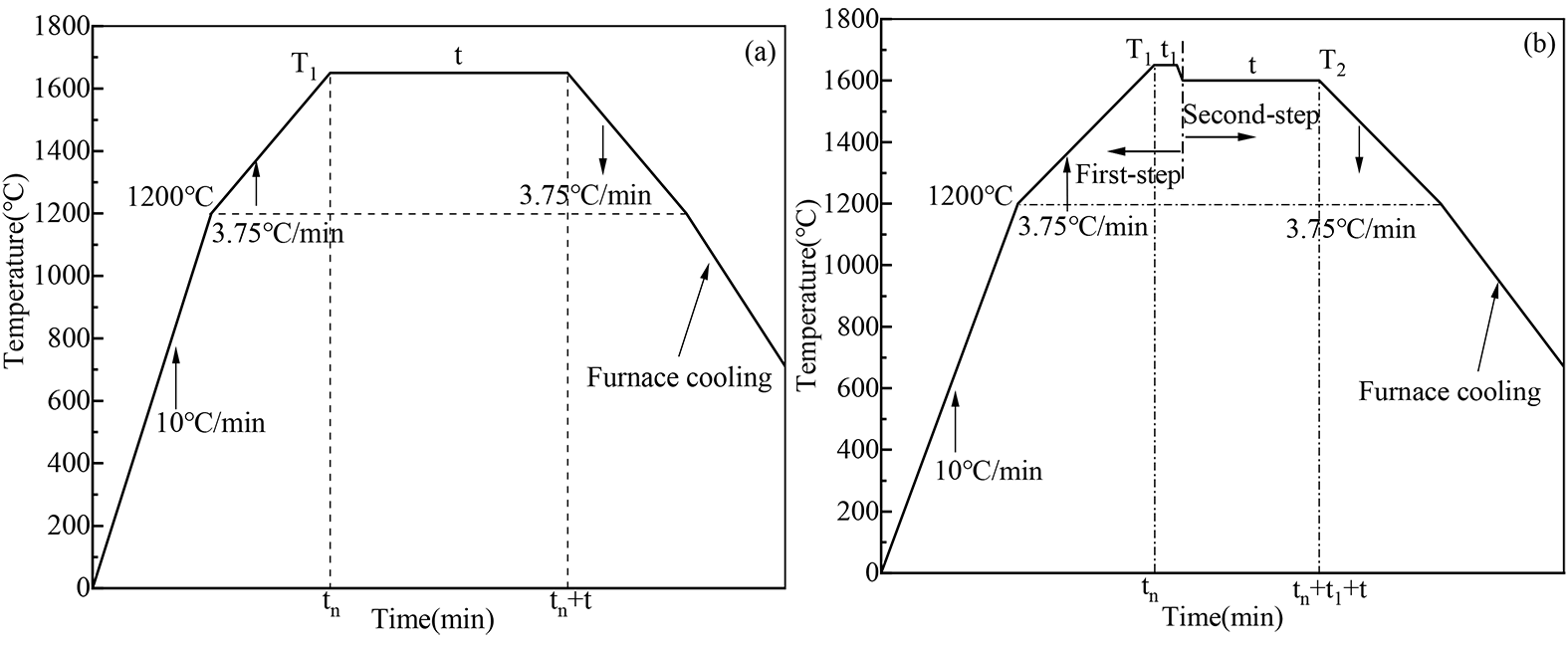
Figure 3a shows the changing curve of sample TS. Firstly, the temperature was raised to 1,200°C at a rate of 10°C/min, then it was increased to T1 at a rate of 3.75°C/min. After that, the temperature was cooled to 1,200°C with a rate of 3.75°C/min. Finally, the temperature was cooled to room temperature. As shown in Figure 3b, the changing curve of TSS is similar to that of TS in the initial stage and then stays briefly after heating to the maximum temperature of T1, and then it is cooled down to T2 at a rate of 50°C/min, and held at t for a certain time. While the other temperature-controlling procedures were the same as that of TS, TSS sintering technology was carried out with two factors and three levels. The specific sintering parameters are shown in Table 2.
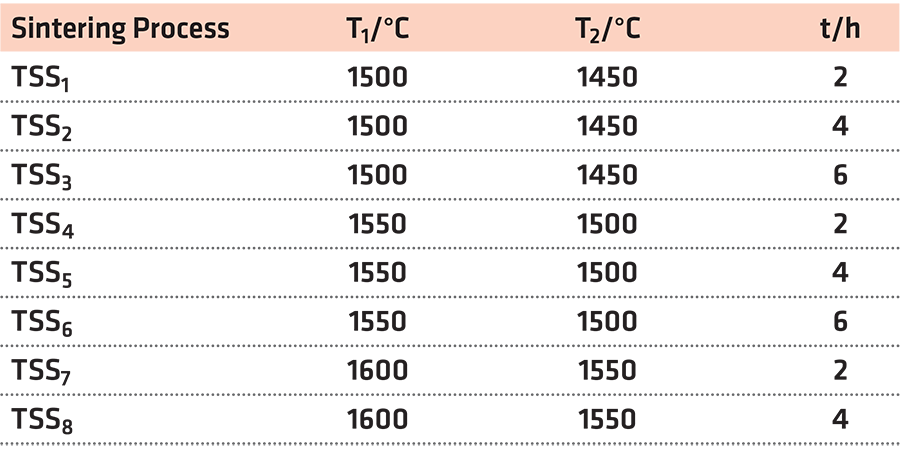
2.4 Characterizations of the Prepared Samples
To evaluate the properties of Al2O3 composite ceramic obtained by various sintering technologies, the ceramic sample was obtained by cutting, polishing with diamond particles, cleaning, drying, and gold spraying. The density was measured using the Archimedes drainage method; the microstructure was characterized using a scanning electron microscope (SEM, Evo18, Zeiss, Oberkochen, BW, Germany), and the grain size was measured using the line intercept method. The hardness, fracture, and toughness were measured using a Vickers hardness tester (HV-1000ZCM-XY, Anyi Instrument Co., Ltd., Shanghai, China), and the three-point bending test was carried out using an electronic universal testing machine (WDW-100KN, Instron Co., Boston, MA, USA). The fracture toughness and bending strength of ceramic specimens were obtained from Equations 1 and 2, respectively.
where P is the pressure load, and C is the average crack length.
where F is the failure load, L is the span, and b and h are the width and thickness of the specimen, respectively.
In addition, the density, particle size, and mechanical properties of the ceramics were characterized using an average of seven tests per sample out of 20 samples from the same batch.
3 Results and Discussion
3.1 Comparison of Results from TSS and TS
The TS and TSS were applied to Al2O3 composite ceramics, and the Drel and AGS of ceramic sintered bodies were obtained, as shown in Figure 4.
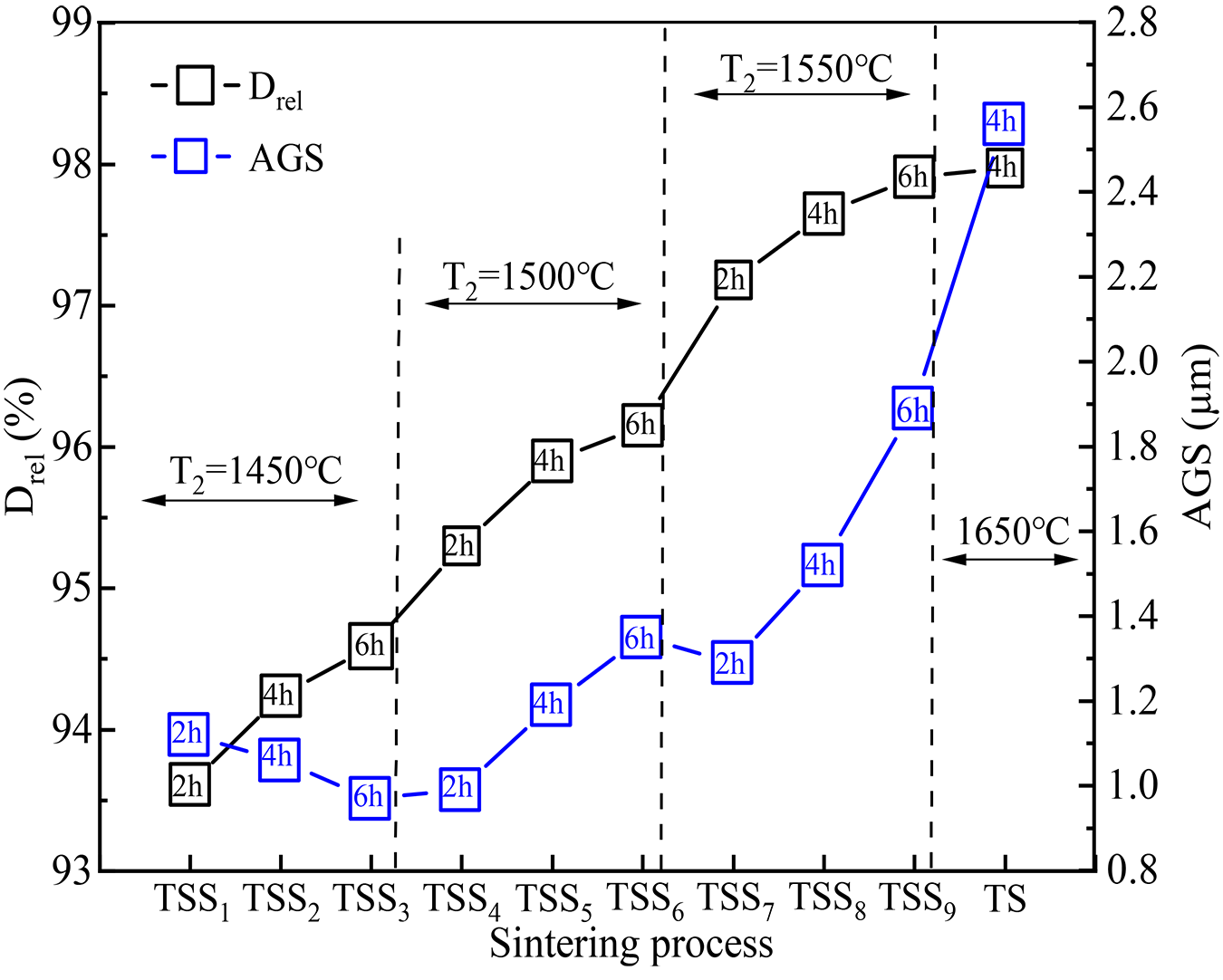
Figure 4 shows the Drel (black mark) and AGS (blue mark) of Al2O3 composite ceramics under the action of each TSS and TS. The same holding time t = 4 h, the Drel (TSS8) (97.65%) obtained via TSS is slightly lower than that of TS (97.97%), but the AGS (TSS8) (1.52 µm) is significantly lower than TS (2.56 µm). With the increase in t to 6 hours, the Drel (TSS9) (97.91%) treated via TSS is close to TS, and the increased AGS (1.89 µm) is still lower than that of TS. Although the Drel of other ceramics (93.61–97.18%) treated with TSS is lower than that of TS, their AGS (1.12–1.29 µm) is significantly lower than that of TS. TSS is more beneficial to Al2O3 composite ceramics from the compactness and grain refinement point of view.
In addition, the Drel and AGS increase the amount of ceramic obtained via TSS with the T2. When t = 2 hours, T2 from 1,450°C increases to 1,550°C, causing the Drel from 93.61% to increase to 97.18% and the AGS from 1.12 µm to increase to 1.52 µm. When the t increases, the change in compactness is not always obvious, and the AGS still grows. At T2 = 1,550°C, t from 2 hours increased to 4 hours, the Drel from 97.18% (TSS7) to 97.65% (TSS8), and the AGS rapidly from 1.29 µm to 1.52 µm. The t increased to 6 hours, the Drel increased to 97.91%, and the AGS increased to 1.89 µm. Slightly lower T2 ensures fine-grained ceramics; the changing compactness leads to different mechanical properties, as shown in Figure 5.
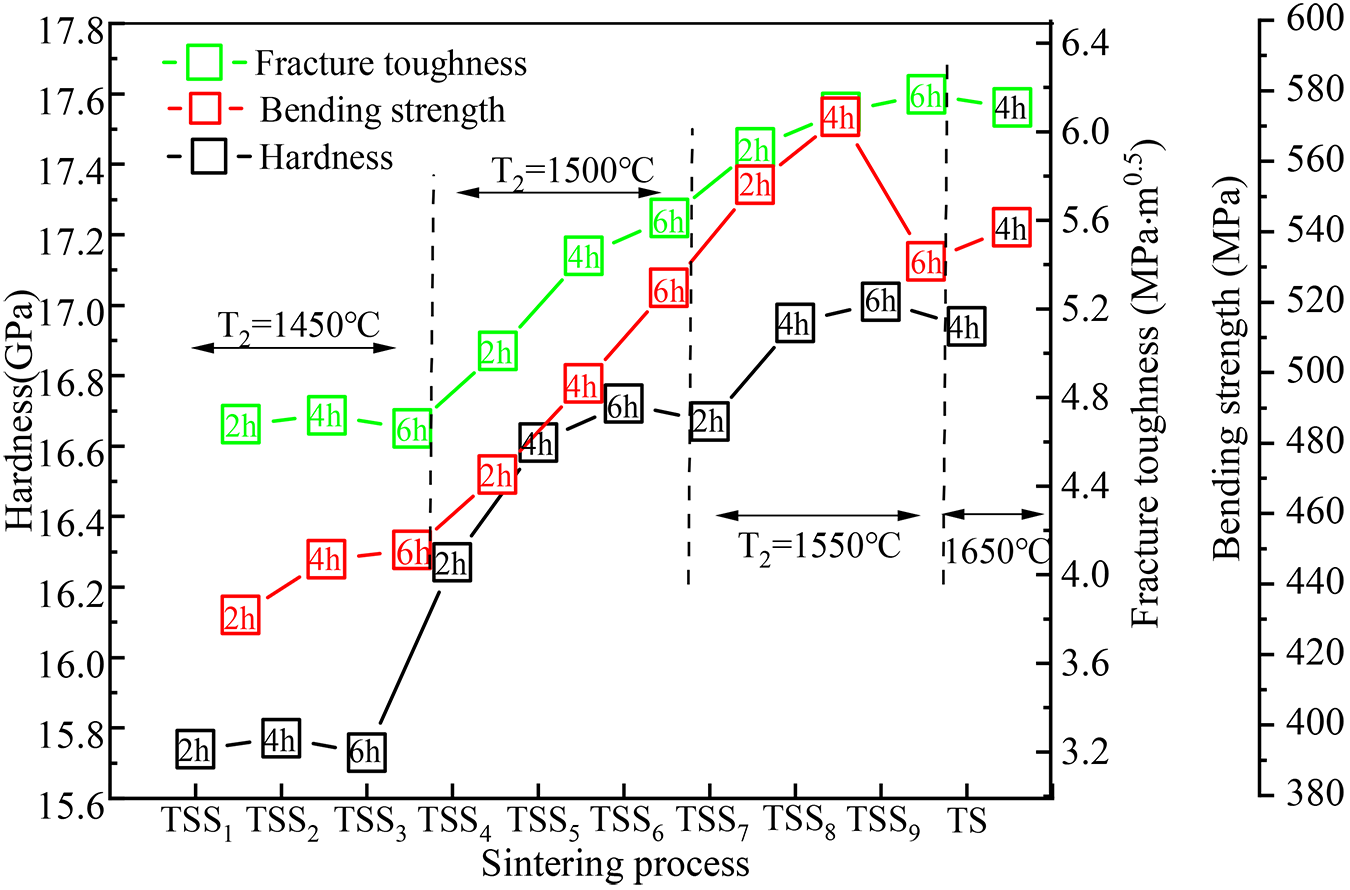
Figure 5 shows the mechanical properties of Al2O3 composite ceramics; the black, red, and green marks correspond to hardness, bending strength, and fracture toughness, respectively. Good ceramic hardness and fracture toughness can be obtained under the sintering conditions of T2 = 1,550°C and t = 6 hours (TSS9), which are 17.01 GPa and 6.17 MPa∙m0.5, respectively. The t was shortened to 4 hours, and the ceramics obtained via TS (16.94 GPa, 6.11 MPa∙m0.5) at 1,650°C were equivalent to TSS8 (16.95 GPa, 6.09 MPa∙m0.5) at 1,550°C. The bending strength obtained via TSS7 (553.34 MPa) at 1550 °C for 2 hours is higher than TS (541.23 MPa).
The mechanical properties of ceramic are improved with the T2. When T2 from 1,450°C to 1,550°C at t = 2 hours, the hardness, bending strength, and fracture toughness increased from 15.74 GPa, 431.14 MPa, and 4.68 MPa∙m0.5 to 16.67 GPa, 553.34 MPa, and 5.93 MPa∙m0.5, respectively. But, the mechanical properties do not improve significantly with the increase in t. For example, the hardness at T2 = 1,550°C, t = 4 hours (TSS8), and t = 6 hours (TSS9) are close, the fracture toughness increases slowly, and the bending strength decreases.
The fine particles of dense ceramics can be obtained under the T2 = 1,550°C, t = 4 hours or 6 hours (Figure 4), and the mechanical properties under the T2 = 1,550°C, t = 4 hours (Figure 5) are close to TS. So, the sintering parameter for obtaining the best Al2O3 composite ceramics is TSS8 with T2 = 1,550°C, t = 4 hours. Using the TSS8 process, the Drel, AGS, hardness, bending strength, and fracture toughness were 97.65%, 1.52 µm, 16.95 GPa, 572.59 Mpa, and 6.09 MPa∙m0.5. The above results preliminarily show that the T2 and t in TSS have varying degrees of effect on the properties of Al2O3 composite ceramics. The impact and reliability of the T2 and t on the properties of ceramics need to be further analyzed through data statistics.
3.2 Effects of T2 and t on Al2O3 Composite Ceramics
The reliability of the impact of factors (T2 and t) on variables (Drel, AGS, and mechanical properties) was evaluated using SPSS software. In the statistical analysis, the adjusted R2 is used to assess the fitting degree of the model, and the standard effect quantity (η2) and p-value are used to evaluate the degree and significance of the effects of T2 and t on ceramic properties, respectively. It is assumed that when p-value < 0.05, the factors have significant effects on variables. On the contrary, the effect of factors on variables is not significant.
3.2.1 Effects of T2 and t on Drel and AGS
The statistical analysis results of T2 and t for Drel and AGS are shown in Table 3, which shows the effects of T2, t, and their interaction (T2 by t) on the Drel of Al2O3 composite ceramics. The adjusted R2 is 0.994, which indicates that the linear regression model has a high degree of fit. The p-value of T2 (0.0016288) and t (0.0029354) is less than 0.05, and the p-value of interaction between both T2 and t (0.6149418) is more than 0.05, which indicates that Drel is only affected by individual T2 and t. Moreover, T2 (η2 = 0.9861565) has a stronger impact on Drel than t (η2 = 0.9639632). Figure 6a shows the interaction between T2 and t for Drel. Figure 6a indicates that there is no interaction between T2 and t because the three kinds of sintering temperatures at holding for 2, 4, and 6 hours are approximately parallel in the t vs. Drel curve.
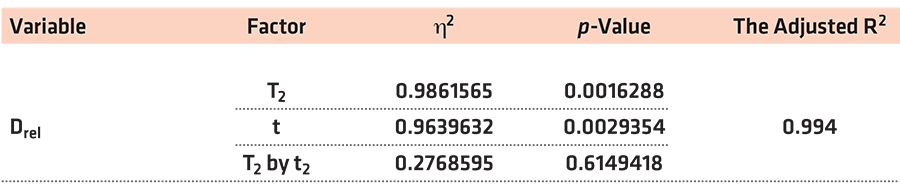
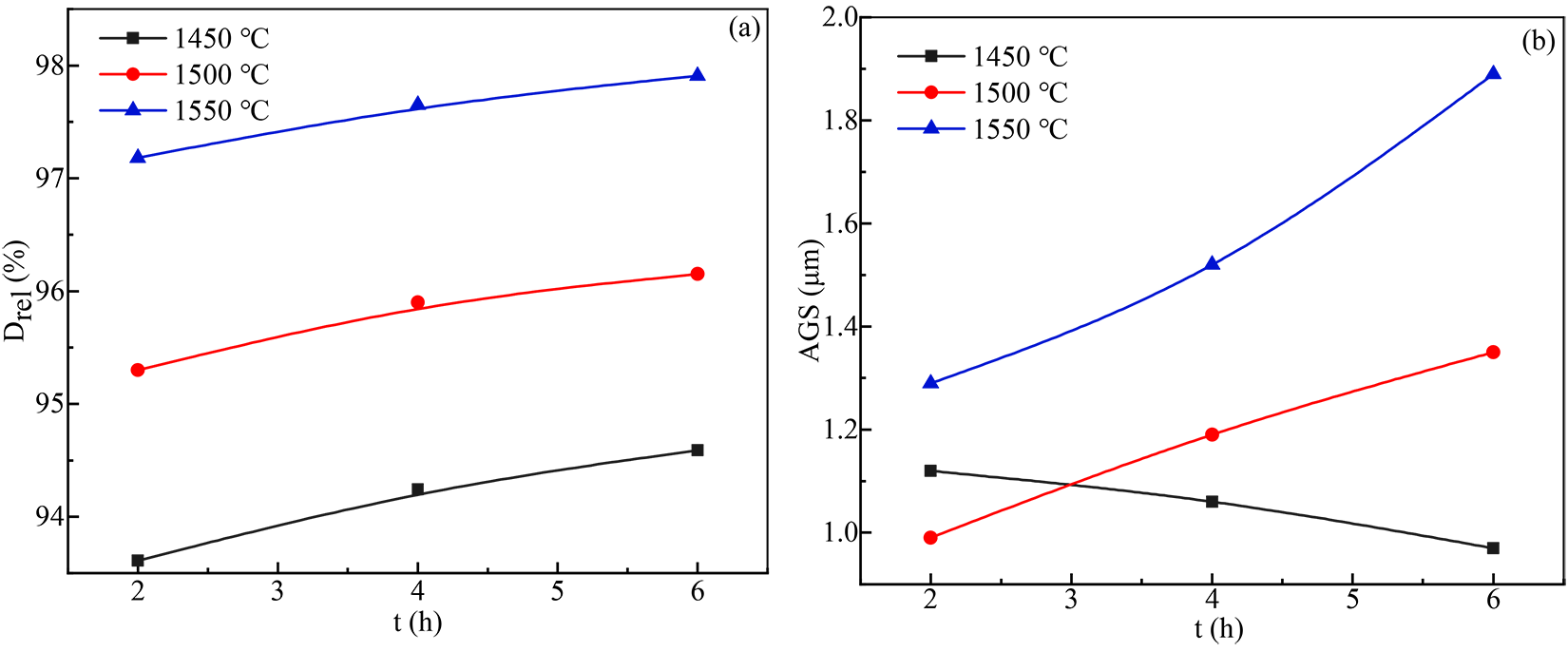
Table 4 shows the effects of T2, t, and their interaction on the AGS of Al2O3 composite ceramics. The adjusted R2 = 0.986 indicates that the static models are valid. The η2 of T2, t, and their interaction gradually rose 0.8967313, 0.9674137, and 0.9755070, and their p-values were less than 0.5. The results show that the above factors had a significant impact on the AGS. However, the interaction of both T2 and t is stronger, followed by T2 and t. Figure 6b shows the interaction between the T2 and t for AGS. Figure 6b indicates there is an interaction between the T2 and t because an intersection of the lines is observed.
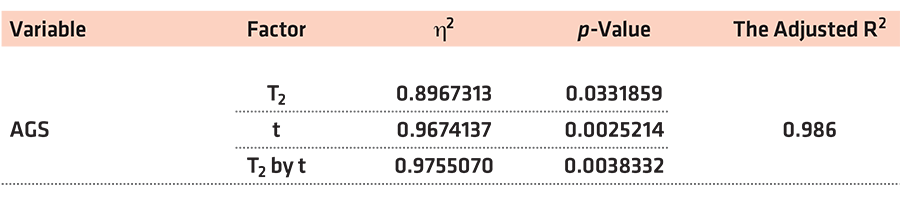
3.2.2 Effects of T2 and t on Mechanical Properties
The T2 and t can be applied to the densification and refinement of Al2O3 composite ceramics to promote the mechanical properties. The statistical analysis of its effect on mechanical properties is shown in Table 5, Table 6, Table 7, and Table 8. The adjusted R2 are 0.978, 0.995, and 0.995 (0.918), respectively, which indicates that the statistic models are suitable.
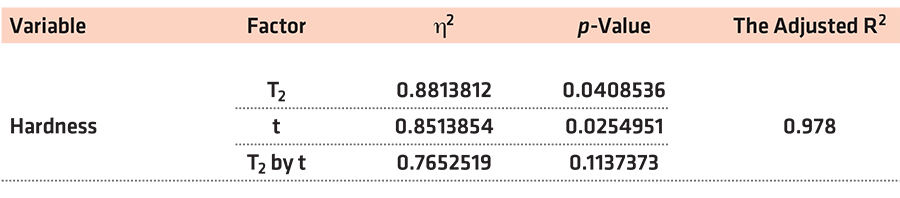
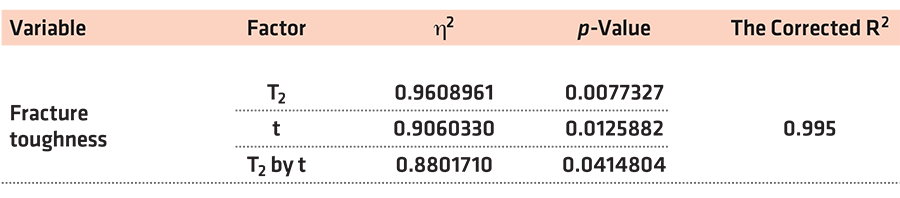
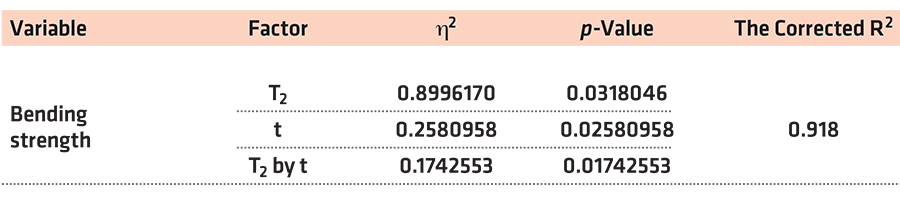
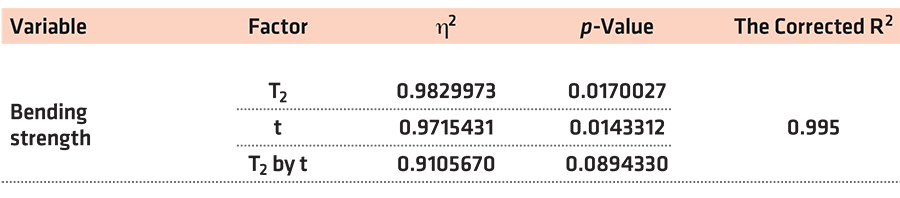
Table 5 presents the impact of T2, t, and their interaction. Both T2 (p-value = 0.0408536) and t (p-value = 0.0254951) significantly affect hardness. However, their interaction is not significant (p-value > 0.05). Moreover, T2 has a stronger impact on hardness than t (0.8813812 against 0.8513854). Figure 7a shows the interaction between the T2 and t for hardness. Figure 7a confirms there is no impact on interaction between T2 and t on hardness because the lines are approximately parallel, as shown in Table 5. In addition, the curve slope is approximately zero at T2 = 1,450°C, which is caused by lower temperature.
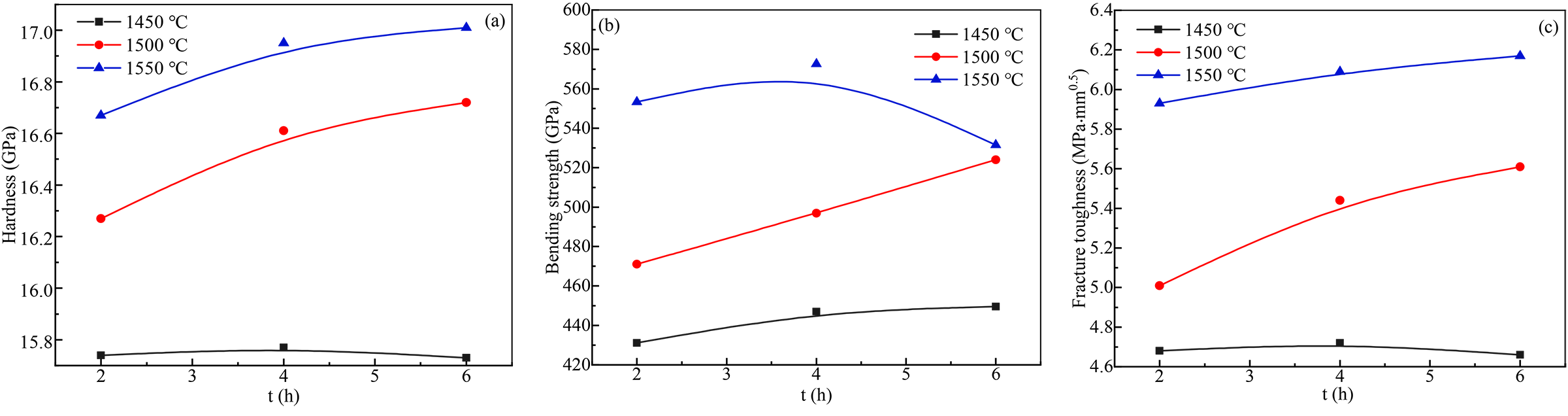
Table 6 presents the impact of T2 and t on fracture toughness. It is observed that T2, t, and their interaction individually affect the fracture toughness and p-values of 0.0077327, 0.0125882, and 0.0414804, respectively. However, the T2 has a more significant effect (η2 = 0.9608961), followed by t and their interaction. Figure 7b shows the interaction between T2 and t for fracture toughness.
Table 7 presents the p-value of T2, which is less than 0.05. It is observed that T2 individually affects bending strength, which obviously contradicts the previous research results (Figure 5). Table 8 shows the impact of T2 and t on bending strength (excluding the data of 1,550°C for 6 hours). It is observed that T2 and t individually affect the bending strength and p-values of 0.0170027 and 0.0143312, respectively. Moreover, Figure 7c shows the curves at T2 = 1,550°C and t ≤ 4 h are almost parallel to each other, and then there is a cross trend at T2 = 1,550°C, t = 6 hours. Therefore, T2 = 1,550°C and t = 6 hours are unsuitable for sintering composite ceramics, which is consistent with the results shown in Figure 4 and Figure 5.
3.2.3 Microstructure of Sintered Ceramics
The performance of Al2O3 composite ceramics depends on the microstructure. This work verifies the advantages of TSS from the micro-level by comparing the microstructure of Al2O3 composite ceramics acted by TS and the TSS8, as shown in Figure 8.
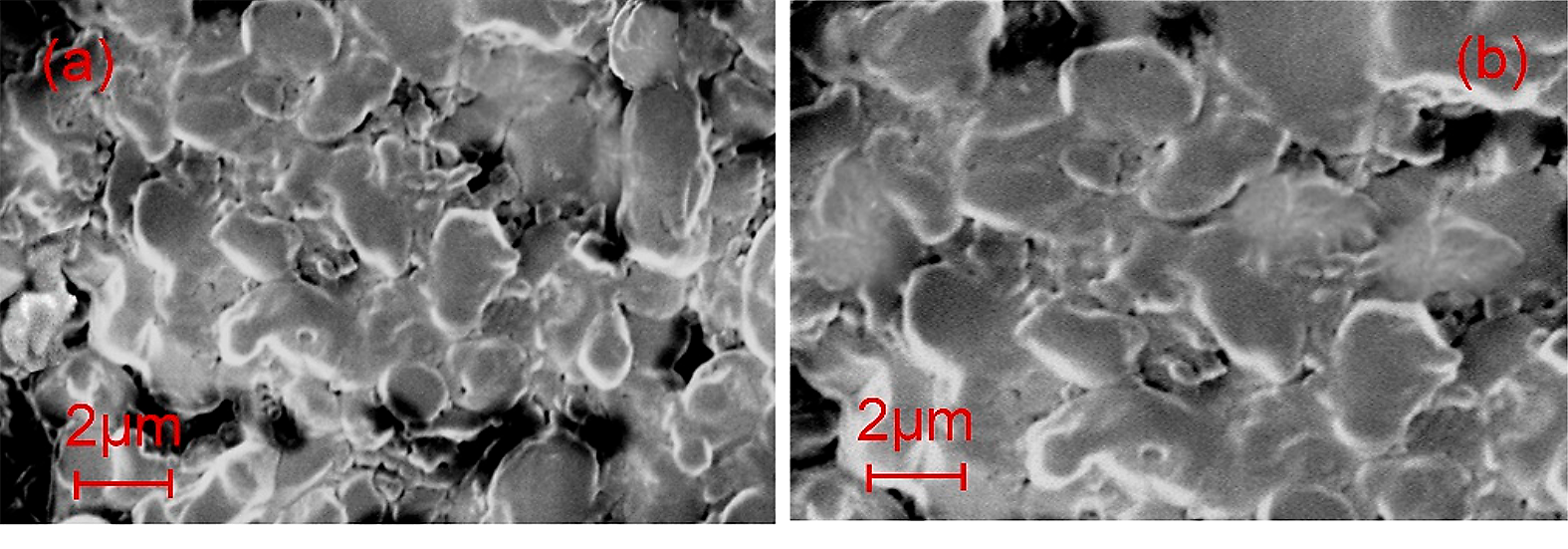
Figure 8a and 8b show the microstructure of Al2O3 composite ceramics obtained via TSS8 at T2 = 1,550°C, t = 4 hours, and TS at 1,650°C, t = 4 hours, respectively. It is found that the ceramic grains obtained via TSS8 are obviously smaller than those obtained via TS. However, there are small gaps between the grains, which results in lower compactness of ceramics obtained by TSS8. The microstructure shown in Figure 8 is consistent with the results of Drel and AGS shown in Figure 4. The above results show the Al2O3 composite ceramics obtained via TSS8 are significantly better than TS; although its compactness is slightly low, the fine-grained ceramics obtained at low cost can bring good comprehensive properties, especially the bending strength and fracture toughness that determine the engineering properties of ceramics.
4 Conclusions
In this work, an Al2O3 composite ceramic was prepared using the MEX-PPM combined process, and the final ceramic samples were obtained via TS and TSS. The effects of sintering processes on Al2O3 composite ceramics were studied using experiments, and the following was concluded:
1. Compared to TS, TSS effectively refined grain size and improved its comprehensive properties. TSS8 can ensure the densification of ceramic before the rapid grain growth; its highest sintering temperature and shortest holding time were T2 = 1,550°C and t = 4 hours, respectively. Under this condition, the Drel and AGS of the ceramics were 97.65% and 1.52 µm. Their hardness, bending strength, and fracture toughness were 16.95 GPa, 572.59 Mpa, and 6.09 MPa·m0.5;
2. Both T2 and t and their interactions individually affect the AGS, fracture toughness, and bending strength significantly, although T2 has more impact. However, both T2 and t affect the Drel and hardness more significantly.
In addition, the microstructure of ceramics obtained via the TSS8 and TS was compared, and the advantages of TSS8 from the microscopic point of view were verified. Commercial Al2O3 ceramics with good fracture toughness and flexural strength via TSS will have unique advantages in engineering applications.
Author Contributions
Conceptualization, X.H. and J.X.; methodology, X.H. and L.H.; formal analysis, X.H., J.X., W.J. and L.H.; writing — original draft preparation, X.H.; writing — review and editing, X.H., W.J. and J.X.; supervision, J.X., L.H. and X.H. All authors have read and agreed to the published version of the manuscript.
Funding
This research was funded by the National Natural Science Foundation of China (Grant No. 51805212), Natural Science Found of Jiangsu Province (Grant No. BK20160182) and the Major Scientific and the Technological Innovation Project of Shandong Province (Grant No. 2019JZZY020111).
Data Availability Statement
Data are available upon request from the corresponding author.
Conflicts of Interest
The authors declare that they have no competing interest.
References
- Medvedovski, E. Wear-resistant engineering ceramics. Wear 2001, 249, 821-828.
- Chen, Z.; Li, J.; Liu, C.; Liu, Y.; Zhu, J.; Lao, C. Preparation of high solid loading and low viscosity ceramic slurries for photopolymerization-based 3D printing. Ceram. Int. 2019, 45, 11549-11557.
- Sun, C.; Tian, X.; Wang, L.; Liu, Y.; Wirth, C.M.; Günster, J.; Li, D.; Jin, Z. Effect of particle size gradation on the performance of glass-ceramic 3D printing process. Ceram. Int. 2017, 43, 578–584.
- Guo, H.; Guo, J.; Baker, A.; Randall, C.A. Hydrothermal Assisted Cold Sintering Process: A New Guidance for Low Temperature Ceramic Sintering. ACS Appl. Mater. Interfaces 2016, 8, 20909-20915.
- Guo, H.; Baker, A.; Guo, J.; Randall, C.A. Protocol for Ultralow-Temperature Ceramic Sintering: An Integration of Nanotechnology and the Cold Sintering Process. ACS Nano 2016, 10, 10606-10614.
- Sofia, D.; Chirone, R.; Lettieri, P.; Barletta, D.; Poletto, M. Selective laser sintering of ceramic powders with bimodal particle size distribution. Chem. Eng. Res. Des. 2018, 136, 536-547.
- Song, S.; Gao, Z.; Lu, B.; Bao, C.; Zheng, B.; Wang, L. Performance optimization of complicated structural SiC/Si composite ceramics prepared by selective laser sintering. Ceram. Int. 2020, 46, 568-575.
- Feng-You, L.; Yu-Ying, L.; Ling, Z.; Xin, S.; Zhi-Hao, Z.; Huan, Z. Effect of TiO2 Sintering Additives on Alumina Ceramic Prepared with Nano-η-Al2O3. J. Synth. Cryst. 2019, 48, 699-704.
- Wen, J.; Wang, H.; Fan, L.; Peng, K.; Su, L. Non-additive sintering of Si2N2O ceramic with enhanced high-temperature strength, oxidation resistance, and dielectric properties. Ceram. Int. 2021, 47, 25689-25695.
- Yan, D.; Xu, X.; Lu, H.; Wang, Y.; Liu, P.; Zhang, J. Fabrication and properties of Y2O3 transparent ceramic by sintering aid combinations. Ceram. Int. 2016, 42, 16640-16643.
- Wu, Z.; Liu, W.; Wu, H.; Huang, R.; He, R.; Jiang, Q.; Chen, Y.; Ji, X.; Tian, Z.; Wu, S. Research into the mechanical properties, sintering mechanism and microstructure evolution of Al2O3-ZrO2 composites fabricated by a stereolithography-based 3D printing method. Mater. Chem. Phys. 2018, 207, 1-10.
- Palmero, P.; Lombardi, M. Influence of the firing parameters on the phase development and microstructural evolution. J. Therm. Anal. Calorim. 2009, 97, 191-196.
- Lóh, N.J.; Simão, L.; Faller, C.A.; De Noni, A.; Montedo, O.R.K. A review of two-step sintering for ceramics. Ceram. Int. 2016, 42, 12556-2572.
- Wang, X.-H.; Chen, P.-L.; Chen, I.W. Two-Step Sintering of Ceramics with Constant Grain-Size, I. Y2O3. J. Am. Ceram. Soc. 2006, 89, 431-437.
- Lukić, M.; Stojanović, Z.; Škapin, S.D.; Maček-Kržmanc, M.; Mitrić, M.; Marković, S.; Uskoković, D. Dense fine-grained biphasic calcium phosphate (BCP) bioceramics designed by two-step sintering. J. Eur. Ceram. Soc. 2011, 31, 19-27.
- Farhandi, H.; Karim, M.N.; Almeida RS, M.; Tushtev, K.; Rezwan, K. Increasing the tensile strength of oxide ceramic matrix mini-composites by two-step sintering. J. Am. Ceram. Soc. 2022, 105, 1928-1938.
- Kim, H.S.; Oh, S.T.; Do Kim, Y. Effects of the two-step sintering process on the optical transmittance and mechanical strength of polycrystalline alumina ceramics. Ceram. Int. 2014, 40, 14471-14475.
- Lin, F.J.T.; Jonghe, L.C.D. Microstructure refinement of sintered alumina by a two-step sintering technique. J. Am. Ceram. Soc. 1997, 80, 2269-2277.
- Bodišová, K.; Šajgalík, P.; Galusek, D.; Švančárek, P. Two-Stage Sintering of Alumina with Submicrometer Grain Size. J. Am. Ceram. Soc. 2007, 90, 330-332.
- Maca, K.; Pouchly, V.; Zalud, P. Two-Step Sintering of oxide ceramics with various crystal structures. J. Eur. Ceram. Soc. 2010, 30, 583-589.
- Li, J.; Ye, Y. Densification and Grain Growth of Al2O3 Nanoceramics During Pressureless Sintering. J. Am. Ceram. Soc. 2006, 89, 139-143.
- Moon, S.M.; Wang, X.; Cho, N.H. Nanostructural and physical features of BaTiO3 ceramics prepared by two-step sintering. Ceram. Soc. Jpn. 2009, 117, 729-731.
- Li, X.; Liu, C.; Sun, B.; Liu, X.; Zuo, Z.; Shu, Y.; Zeng, X.; Yi, J.; Chen, H.; Liu, Y.; et al. Refined grain size of ITO ceramic targets prepared by pressure slip casting and two-step sintering. J. Eur. Ceram. Soc. 2021, 4, 3501-3511.
- Hong, D.; Yin, Z.; Yan, S.; Xu, W. Fine grained Al2O3/SiC composite ceramic tool material prepared by two-step microwave sintering. Ceram. Int. 2019, 45, 11826-11832.
- Razavi Hesabi, Z.; Haghighatzadeh, M.; Mazaheri, M.; Galusek, D.; Sadrnezhaad, S.K. Suppression of grain growth in sub-micrometer alumina via two-step sintering method. J. Eur. Ceram. Soc. 2009, 29, 1371-1377.
- Lóh, N.J.; Simão, L.; Jiusti, J.; De Noni, A.J.; Montedo OR, K. Effect of temperature and holding time on the densification of alumina obtained by two-step sintering. Ceram. Int. 2017, 43, 8269-8275.
- Lóh, N.J.; Simão, L.; Jiusti, J.; Arcaro, S.; Raupp-Pereira, F.; De Noni, A.; Montedo, O.R.K. Densified alumina obtained by two-step sintering: Impact of the microstructure on mechanical properties. Ceram. Int. 2020, 46, 12740-12743.
- He, X.; Xu, J.; Ji, W. The effect of surfactants on the performances of ceramic slurry by material extrusion and photo-polymerization combined molding process. J. Ceram. Soc. Jpn. 2021, 129, 489-495.
- Zhou, M.P.; Liu, W.; Wu, H.D.; Song, X.; Chen, Y.; Cheng, L.X.; He, F.P.; Chen, S.X.; Wu, S.H. Preparation of a defect-free alumina cutting tool via additive manufacturing based on stereolithography-Optimization of the drying and debinding processes. Ceram. Int. 2016, 42, 11598-11602.
- He, X.; Xu, J.; Ji, W. Effects of n-Al2O3 and µ-TiCN on Microstructure and Mechanical Properties of Al2O3 Composite Ceramics Manufactured by Material Extrusion and Photo-Polymerization Combined Process. Crystals 2022, 12, 745.
- Liu, Z.; Bhandari, B.; Prakash, S.; Mantihal, S.; Zhang, M. Linking rheology and printability of a multicomponent gel system of carrageenan-xanthan-starch in extrusion based additive manufacturing. Food Hydrocoll. 2019, 87, 413-424.
© 2023 by the authors. Licensee MDPI, Basel, Switzerland. This article is an open access article (https://www.mdpi.com/2073-4352/13/12/1679) distributed under the terms and conditions of the Creative Commons Attribution (CC BY) license (https://creativecommons.org/licenses/by/4.0/). This article has been edited to conform to the style of Thermal Processing magazine.